Heart Rate and Blood Pressure
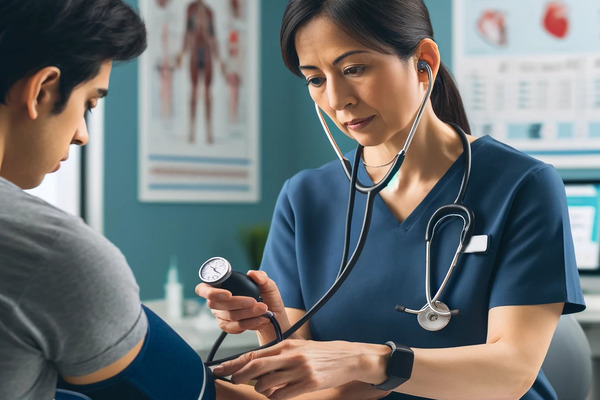
Heart rate and blood pressure play pivotal roles in the circulatory system, ensuring blood is properly distributed throughout the body to meet physiological demands. Adjustments in heart rate and blood pressure occur in response to changes in exercise intensity, body position, the mode of exercise, and states of arousal. Although they can function independently, these factors are often interrelated, with changes in one frequently leading to adjustments in the other.
Heart rate serves as a crucial indicator of cardiorespiratory function. At rest, a typical heart rate ranges from 60 to 80 beats per minute, but this can decrease significantly in well-trained athletes due to enhanced stroke volume and increased parasympathetic nervous system influence. Conversely, a high resting heart rate may indicate poor cardiorespiratory health or factors like overtraining and stress. Monitoring heart rate, both at rest and during exercise, helps in adjusting and individualizing training programs, making heart rate monitors invaluable tools for athletes and coaches alike.
The sinoatrial (SA) node, the heart’s natural pacemaker located on the right ventricle, initiates the heart’s impulse. This node, regulated by both sympathetic and parasympathetic nerve fibers from the medulla oblongata and central nervous system’s cardiorespiratory control centers, controls heart rate. Sympathetic fibers increase heart rate, while parasympathetic fibers decrease it. During exercise, a reduction in parasympathetic influence and an increase in sympathetic activity elevate heart rate to accommodate increased circulatory demands.
Blood pressure fluctuates to supply the necessary blood flow for various activities, making it essential for trainers to understand blood pressure dynamics. Despite its significance, blood pressure is not typically used as a fitness indicator, though trainers should be aware of normal ranges and factors influencing blood pressure changes.
Resting heart rate measurements should be taken under consistent conditions to accurately reflect the heart’s parasympathetic influence. This involves measuring heart rate early in the morning, in a relaxed state, and in a distraction-free environment. Tracking changes in resting heart rate over time can provide insights into an athlete’s training adaptations and overall cardiovascular health.
Together, heart rate and blood pressure adjustments are essential for meeting the circulatory demands of the body during different physiological states, highlighting the importance of monitoring these factors in both health and disease for optimizing athletic performance and training.
Exercise Intensity and Heart Rate
Heart rate (HR) is a critical measure of cardiorespiratory function, directly correlating with exercise intensity and oxygen consumption. Its importance is underscored in numerous cardiorespiratory fitness tests, which estimate oxygen consumption based on steady-state heart rate (SSHR) at specific workloads. When the circulatory system meets the demands of an activity, resulting in a stable HR, it signifies SSHR, making HR a reliable indicator of the exertion level for that activity.
Maximal heart rate (MHR), the peak number of heart beats per minute achieved during maximal effort, generally decreases with age and is not significantly influenced by cardiorespiratory training improvements. Age-predicted maximal heart rate (APMHR) is commonly calculated by subtracting one’s age from 220, serving as a guideline for establishing HR upper limits during physical activity without requiring maximal effort testing.
Heart rate reserve (HRR) builds on the concept of APMHR, integrating resting heart rate (RHR) to tailor exercise intensity guidelines. The Karvonen formula, a widely recognized method for determining training intensity, calculates HRR by subtracting RHR from APMHR. A percentage of this reserve, added to RHR, establishes a target HR for training, allowing for adjustments based on the individual’s fitness level or training goals.
Active HR monitoring, particularly with recent advancements in heart rate monitors, enables precise training adjustments and the assessment of chronic adaptations in athletes. Changes in RHR and exercise HR at specific intensities provide insights into cardiorespiratory efficiency, making HR an indispensable tool in health and fitness industries. The versatility of HR measurements, from resting conditions to exercise, offers a noninvasive approach to gauge exercise intensity and cardiorespiratory fitness across varying levels of physical conditioning.
The efficiency of the cardiorespiratory system is evident in how HR adjusts between different workloads, with trained individuals demonstrating more efficient HR responses compared to sedentary counterparts. This efficiency is further reflected in the quicker normalization of HR post-exercise in trained individuals. Understanding the dynamics of HR and blood pressure adjustments in response to physical demands highlights the interconnectedness of these circulatory factors and their role in maintaining optimal physiological function during various activities.
Sport Performance and Heart Rate
Monitoring heart rate (HR) can effectively control and optimize a training regimen and its adaptations. By adjusting the training intensity to challenge yet remain sustainable for the athlete’s current cardiorespiratory efficiency, HR monitoring aids in progressively improving fitness. The Karvonen method, in particular, offers a personalized approach by setting specific upper and lower HR limits, thereby keeping the athlete engaged and ensuring a focused intensity level. This approach not only motivates the athlete but also provides coaches with objective insights into workout difficulties and athlete conditions over time.
For those with varying fitness levels, establishing a target heart rate as a percentage of the age-predicted maximal heart rate (APMHR) might seem rudimentary. However, this method uniquely tailors exercise intensity since individuals require different levels of effort to reach the same HR targets. Thus, what appears to be a generic formula actually adapts to each person’s fitness status, allowing for personalized training intensities.
Target HRs facilitate varied training intensity within a workout or across a training season, accommodating the dynamic needs of athletes like soccer midfielders, who require both endurance and the ability to perform high-intensity sprints. Long endurance sessions might target a moderate intensity, monitored through HR to ensure the pace aligns with cardiorespiratory gains, thereby indicating when to increase speed for further fitness enhancements. During high-intensity interval training, HR monitoring aids in gauging recovery between sprints, allowing trainers to tailor recovery intervals for customized challenges or to simulate game-like conditions, ultimately enhancing performance.
This multifaceted approach underscores the utility of HR monitoring in adjusting training intensity, evidencing cardiorespiratory improvements, and personalizing athlete training programs to foster continuous progression and peak performance.
Heart Rate Measurement
Numerous methods exist for measuring heart rate (HR), including palpation, auscultation, Doppler ultrasonic monitoring, and electrical monitoring, with electrocardiography (ECG) regarded as the gold standard. Here’s an overview of the most commonly used techniques in nonclinical settings:
Palpation involves feeling the pulse through the distension of arteries as blood passes through. The radial artery on the wrist and the carotid artery are frequent palpation sites. While palpation is a straightforward method, accuracy can be compromised by factors like excessive adipose tissue, movement during measurement, and incorrect application of pressure, especially on the carotid artery.
Procedure for Palpation:
- Begin counting the first detected pulsation as zero, then count the number of beats within a set time frame.
- Commonly, pulses are monitored for time periods that are easily convertible to a minute (e.g., 10, 15, or 30 seconds).
- Another method involves counting 30 beats, measuring the time taken, and dividing 1,800 by the seconds required for 30 beats to estimate bpm.
Auscultation replaces the felt pulse waves with the sounds produced by heart contractions, heard through a stethoscope placed over the heart’s apex or base.
Procedure for Auscultation:
- Position the stethoscope’s diaphragm flush against the skin over the heart, applying slight pressure to enhance sound quality.
- Count or time the heartbeats similarly to palpation, noting that both methods may be easier to perform during exercise due to stronger heart contractions.
Electrocardiography (ECG) captures the heart’s electrical activity through a coordinated wave of ions across cardiac tissue, depicted in a P wave, QRS complex, and T wave on an ECG waveform. The R wave, signifying ventricular contraction, is typically used for HR calculation in healthy individuals.
Procedure for Calculating HR Using an ECG Strip:
- Identify two consecutive R waves on the ECG strip.
- Count the number of small boxes between these waves and divide 1,500 by this count to determine HR.
Monitoring HR is a vital component of managing exercise intensity, enabling control over training regimens for optimized adaptation. The Karvonen method is a refined approach that calculates exercise intensity by establishing a target HR zone based on age-predicted maximal heart rate (APMHR) and resting heart rate (RHR), factoring in the individual’s fitness level to tailor workout intensity.
These HR measurement techniques offer various degrees of accuracy and applicability, with ECG providing precise measurements under both resting and active conditions. Together, they provide essential tools for fitness practitioners to gauge cardiovascular efficiency, adjust training intensity, and monitor progress over time, accommodating the unique requirements of athletes across different levels of physical conditioning.
Heart Rate Monitors
Monitoring heart rate (HR) is essential for understanding cardiorespiratory performance both at rest and during exercise. The advancement in personal HR monitoring devices has notably improved their utility in tracking exercise intensity. These devices, functioning on principles similar to electrocardiogram (ECG) machines, use small electrodes embedded in a reusable chest strap to detect the heart’s electrical impulses. These impulses are transmitted to a wrist-worn digital display, offering real-time HR data, with the mean HR being recalculated and updated approximately every five seconds. This provides an accurate, objective measure of relative exercise intensity.
Further enhancing their utility, more sophisticated HR monitors can connect to computer programs, allowing for the longitudinal tracking of training HR. This feature is invaluable for athletes and fitness enthusiasts aiming to optimize their training regimens through detailed analysis of their cardiorespiratory output over time.
Given the importance of HR monitoring in assessing cardiorespiratory function, acquiring and refining HR monitoring techniques should be a priority for exercise professionals. Regular practice of these techniques ensures proficiency in using HR data to guide training decisions, making HR monitors an integral part of a comprehensive exercise management strategy.
Blood Pressure
Blood pressure (BP) is a critical measure within the cardiovascular system, reflecting the force exerted by circulating blood on vessel walls. It’s maintained by a complex interplay of factors such as vessel elasticity, resistance to blood flow, ventricular contractions, along with blood volume and viscosity. BP varies throughout the day influenced by metabolic demands, body position, arousal, diet, and several other factors, with hormonal, hemodynamic, and anatomical aspects working in concert to ensure adequate circulation.
Understanding BP control and assessment is essential in health promotion and healthcare, given its role as a key vital sign for health evaluation. Normal resting systolic BP ranges between 100 and 120 mmHg, while diastolic BP ranges between 75 and 85 mmHg. Deviations from these ranges can indicate hypertension (chronically elevated BP) or hypotension (abnormally low BP), each posing distinct health risks. During physical exertion, BP adjustments are necessary to meet increased circulatory demands.
Hypertension is a prevalent cardiovascular risk factor, often going undetected due to its asymptomatic nature. Despite the elusive cause of primary hypertension, diagnosis and treatment are relatively straightforward, highlighting the importance of regular BP monitoring. Since BP can fluctuate, accurate diagnosis requires monitoring at various times, potentially utilizing a 24-hour BP monitor to capture real-life variations and mitigate “white coat syndrome.”
Conversely, hypotension indicates insufficient systemic pressure to satisfy circulatory demands, characterized by systolic readings under 90 mmHg and diastolic readings under 60 mmHg. This condition can result in inadequate blood flow to vital organs, with symptoms like dizziness, disorientation, or even fainting. Causes of hypotension vary, including dehydration, medication effects, and other health issues. Preventive measures focus on hydration, limiting alcohol intake, and avoiding prolonged standing.
Overall, the physiological regulation and measurement of BP are fundamental for detecting, understanding, and managing conditions that affect cardiovascular health. Both hypertension and hypotension warrant careful monitoring and management to mitigate their potential impact on well-being.
Pressure Gradients and Blood Pressure
Blood pressure (BP) within the circulatory system is determined by a myriad of factors working in unison to create the necessary pressure gradients (PG) for blood circulation across various physiological states. This pressure, essential for the distribution of blood, fluctuates in response to metabolic demands, body position, arousal levels, and other variables. BP’s control mechanisms involve the interplay of vessel elasticity, resistance to blood flow, ventricular contractions, blood volume, and viscosity, ensuring adequate blood flow through the arteries, capillaries, and veins.
The arterial blood pressure (ABP) varies along the cardiovascular loop, with its highest and lowest points being the systolic blood pressure (SBP) and diastolic blood pressure (DBP), respectively. Pulse pressure (PP) represents the difference between these two pressures, serving as an indicator of arterial compliance at rest and adjusting during vigorous activities to accommodate increased blood flow needs. Mean arterial pressure (MAP), representing the average arterial pressure, is calculated differently during rest and exercise to reflect changes in the cardiac cycle’s phases due to increased heart rates during physical activity.
Regulation of ABP involves adjusting blood volume within the arterial system through mechanisms that alter cardiac output, total peripheral resistance (TPR), or both. Cardiac output, the volume of blood ejected from the left ventricle per minute, and TPR, the opposition to blood flow through vessels, are key factors in this regulation. Acute changes in ABP are monitored by baroreceptors located in the aortic arch and carotid arteries, which send feedback to the central nervous system to adjust BP accordingly.
Hypertension, a prevalent cardiovascular risk factor, is characterized by chronically elevated BP, posing significant health risks, whereas hypotension indicates dangerously low BP levels, potentially leading to circulatory shock. Monitoring BP is crucial for identifying these conditions, with 24-hour BP monitors offering insights into BP patterns throughout daily activities and helping to avoid misdiagnoses due to situational factors like white coat syndrome.
The development and maintenance of PGs facilitate blood movement from areas of higher pressure to lower pressure, essential for the circulatory system’s function. From the heart’s contractions and relaxations creating initial PGs to the venous mechanisms ensuring blood’s return to the heart, the body’s anatomical and physiological features are intricately designed to support this dynamic process. Understanding these mechanisms is fundamental for health professionals to promote cardiovascular health and manage conditions like hypertension and hypotension effectively.
Exercise and Arterial Blood Pressure Regulation
Blood pressure (BP) regulation and changes during exercise involve complex physiological responses that ensure adequate blood flow to meet tissue demands. The arterial blood pressure (ABP) is particularly influenced by various factors, including systolic blood pressure (SBP) and diastolic blood pressure (DBP), which adjust to exercise intensity and the metabolic requirements of tissues. During aerobic exercise, SBP typically rises to accommodate the increased demand for oxygen and nutrients, while DBP remains relatively stable, expanding mean arterial pressure (MAP) and pulse pressure (PP). This adjustment involves a sympathetic response, releasing epinephrine and norepinephrine, which increases heart rate (HR) and stroke volume (SV), consequently boosting ABP and facilitating enhanced blood distribution to active tissues.
When engaging in activities such as cycling, the intensity of the exercise dictates the blood pressure response. Flat surface cycling requires a modest BP increase, whereas cycling uphill demands a significant ABP rise to ensure sufficient blood flow to heavily engaged leg muscles. Prolonged, vigorous activities that induce sweating can lead to dehydration, reducing plasma volume, elevating hemoconcentration, and potentially lowering BP. In such scenarios, the antidiuretic hormone (ADH) is secreted to conserve water and mitigate hemoconcentration.
Anaerobic exercises like weight lifting cause notable increases in both SBP and DBP, with pressures soaring during maximal lifts, which poses considerations for individuals with cardiovascular conditions. The American Heart Association notes the safety and benefits of strength training when guidelines are adhered to, despite the potential risks associated with high BP spikes during such activities. These spikes are particularly pronounced during efforts involving the Valsalva maneuver, which stabilizes the core but can dangerously elevate BP.
Long-term BP regulation involves the kidneys and various hormones that manage fluid balance within the body. Fluid conservation or excretion by the kidneys plays a pivotal role in maintaining BP stability. The renin-angiotensin-aldosterone system is a key regulatory mechanism, with angiotensin II acting as a potent vasoconstrictor and aldosterone promoting sodium reabsorption to retain fluids. This system intricately balances TPR and extracellular fluid volume to regulate arterial blood volume and pressure effectively.
Understanding the dynamics of BP, particularly in response to acute and chronic physiological changes, is essential for managing health and optimizing physical performance. Whether through aerobic or anaerobic exercise, the body’s adaptive mechanisms work to ensure circulatory efficiency, highlighting the importance of managing hydration, exercise intensity, and long-term cardiovascular health through lifestyle and exercise choices.
Arterial Blood Pressure Measurement
Measuring arterial blood pressure (ABP) has evolved from early methods using water columns to more compact and manageable mercury columns, and today, ABP is universally reported in millimeters of mercury (mmHg). However, due to the toxicity of mercury, many health professionals have transitioned to using automated BP cuffs or aneroid devices, which can offer high accuracy if regularly calibrated.
ABP can be measured invasively via indwelling catheters in clinical settings, but more commonly, a sphygmomanometer is used in nonclinical environments. This cuff method temporarily occludes blood flow through the brachial artery, allowing a technician to listen for Korotkoff sounds as air pressure is released from the cuff, indicating SBP and DBP.
The procedure for measuring ABP involves preparing the subject, positioning the cuff and stethoscope correctly, and inflating the cuff before gradually releasing the pressure to listen for the Korotkoff sounds, which mark the SBP and the point at which normal blood flow resumes, indicating DBP. Accurately measuring ABP requires following standardized procedures, although it typically doesn’t require extensive medical training.
Resting heart rate, a measure of fitness, generally indicates better cardiovascular health when lower. Yet, it’s important to note that both trained athletes and untrained individuals can have varying resting heart rates due to a multitude of factors, including stress, caffeine, medications, and overtraining. Monitoring resting heart rate can provide insight into potential health issues or the need to adjust training intensity.
Heart rate monitoring serves as a crucial tool in training, offering objective data on an individual’s physiological response to exercise. This is particularly useful in managing exercise intensity and identifying when an athlete may be pushing beyond their limits, which could be masked by their subjective reporting. The advent of heart rate monitors has enhanced this process, offering features like pacing alerts and the ability to track and analyze training sessions over time.
As exercise intensity increases, such as during cycling up a hill or during intense anaerobic activity like weight lifting, both SBP and DBP can rise significantly to supply the muscles with sufficient oxygen. These activities can cause ABP to adjust to meet metabolic demands, with mechanisms like the release of sympathetic neurotransmitters and temporary vasoconstriction playing key roles.
Long-term regulation of ABP involves the kidneys and hormones in managing fluid balance and responding to fluid losses or surpluses. The renin-angiotensin-aldosterone system, for instance, plays a crucial role in adjusting TPR and blood volume to maintain or adjust ABP as needed.