Exercise Endocrinology
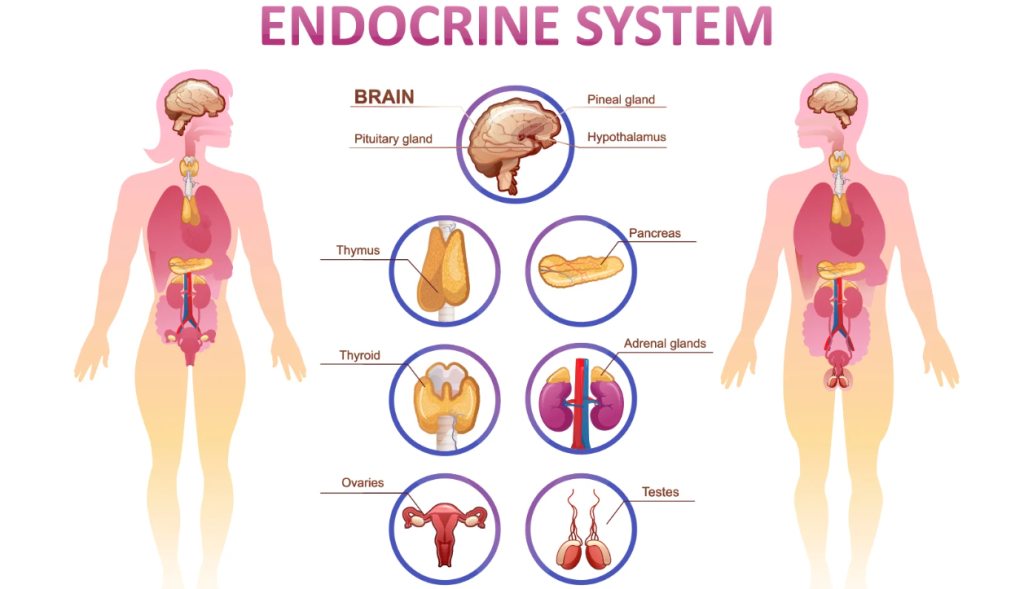
Sports endocrinology is a specialized field that explores the complex interplay between hormones and physical activity. Understanding how hormones regulate various physiological processes during exercise is crucial for optimizing athletic performance, enhancing training adaptations, and promoting overall health.
The endocrine system comprises a network of glands that produce and release hormones into the bloodstream, where they travel to target organs and tissues to regulate numerous functions. Key glands include the pituitary, thyroid, adrenal glands, pancreas, and gonads (ovaries and testes), each secreting specific hormones essential for metabolism, growth, stress response, and reproductive functions.
Hormones in Exercise Physiology:
Insulin and Glucagon: Research has shown that insulin facilitates glucose uptake into cells during exercise, crucial for maintaining blood glucose levels and supporting muscle contraction (Hawley et al., 2018). Glucagon, on the other hand, plays a role in promoting glycogen breakdown in the liver, ensuring a steady supply of glucose during prolonged physical activity (Felig & Wahren, 1975).
Catecholamines (Epinephrine and Norepinephrine): Studies indicate that catecholamines enhance lipolysis and glycogenolysis, contributing to increased energy availability and metabolic rate during exercise (Forsythe et al., 1988). These hormones facilitate the mobilization of energy substrates to active muscles, optimizing performance.
Cortisol: Research findings suggest that cortisol levels rise during exercise to mobilize energy reserves through gluconeogenesis and protein breakdown, supporting sustained physical exertion (Viru, 1992). However, chronic elevations in cortisol due to overtraining may lead to negative health outcomes and performance decrements.
Growth Hormone: Studies have demonstrated that growth hormone secretion increases with exercise intensity, promoting muscle protein synthesis, tissue repair, and fat metabolism (Godfrey et al., 2003). This hormone plays a crucial role in muscle growth and adaptation to resistance training.
Sex Hormones (Testosterone and Estrogen): Research highlights the role of testosterone in muscle protein synthesis and strength gains during resistance training (Ahtiainen et al., 2016). Estrogen influences bone health and metabolic stability, supporting endurance capacity and cardiovascular function in female athletes (Ekenros et al., 2011).
Understanding the roles of these hormones in exercise physiology is essential for tailoring training programs to optimize performance and support long-term health outcomes.
Hormonal Responses to Exercise
Exercise elicits dynamic hormonal responses tailored to meet the metabolic demands imposed by physical activity. The type, intensity, and duration of exercise influence the magnitude and nature of hormonal changes:
Endurance Exercise:
Catecholamines and Cortisol: Prolonged aerobic activities such as running or cycling elevate catecholamines and cortisol levels. These hormones mobilize energy substrates like glucose and fatty acids, supporting sustained muscle contraction and endurance performance.
Resistance Training:
Testosterone and Growth Hormone: High-intensity resistance exercises stimulate testosterone and growth hormone release, promoting muscle protein synthesis, hypertrophy, and strength gains. These hormonal adaptations are crucial for enhancing muscular power and performance.
Exercise Intensity: Higher exercise intensities typically result in more pronounced hormonal responses, optimizing energy mobilization and metabolic adaptations to meet increased physiological demands.
Research has shown that hormonal responses vary based on exercise modality and individual training status, influencing performance outcomes and adaptive responses (Kraemer et al., 2005). Understanding these hormonal responses helps athletes and coaches design effective training protocols that maximize performance gains while minimizing the risk of overtraining and injury.
Insulin and Glucagon Adaptation in Exercise
Insulin and glucagon are pivotal hormones that regulate glucose metabolism, ensuring energy availability during exercise. Understanding their roles, adaptations, and interactions is crucial for optimizing athletic performance, metabolic health, and exercise-related physiological responses.
Insulin
Insulin is a peptide hormone secreted by the beta cells of the pancreas in response to elevated blood glucose levels. Its primary role is to facilitate glucose uptake into cells, promoting energy storage as glycogen in the liver and muscles, and inhibiting gluconeogenesis (the production of glucose from non-carbohydrate sources).
Role in Exercise:
Glucose Uptake: During exercise, insulin secretion decreases to enhance glucose delivery to active muscles. This process promotes glycogen synthesis and supports ATP production, crucial for muscle contraction and sustained physical exertion (Richter & Hargreaves, 2013).
Muscle Anabolism: Insulin facilitates amino acid uptake and protein synthesis, supporting muscle repair and adaptation to exercise-induced damage (Ivy, 1997).
Recovery: Post-exercise, insulin sensitivity increases, enhancing glucose uptake and glycogen replenishment, promoting recovery and muscle glycogen resynthesis (Felig & Wahren, 1975).
Glucagon
Glucagon is a peptide hormone synthesized by alpha cells of the pancreas. It acts antagonistically to insulin, mobilizing glucose from liver glycogen stores (glycogenolysis) and promoting gluconeogenesis to maintain blood glucose levels during fasting or exercise.
Role in Exercise:
Glycogenolysis: Glucagon secretion increases during exercise to stimulate glycogen breakdown in the liver, releasing glucose into the bloodstream for energy production in active muscles (Jensen et al., 1988).
Energy Mobilization: Glucagon supports lipid metabolism and ketone body production, providing alternative energy substrates during prolonged physical exertion (Sherwin & Sacca, 1984).
Regulatory Balance: The insulin-glucagon axis maintains glucose homeostasis during exercise, ensuring adequate energy supply and metabolic stability across varying intensities and durations of physical activity.
Hormonal Responses to Exercise
Endurance Exercise
Endurance exercise, such as running or cycling, elicits dynamic hormonal responses to support prolonged physical exertion and energy metabolism:
Insulin: Insulin levels decrease during moderate to high-intensity endurance activities, facilitating glucose uptake into active muscles and supporting glycogen utilization for sustained energy production (Hawley et al., 2018).
Glucagon: Conversely, glucagon secretion increases to promote glycogenolysis and gluconeogenesis, ensuring a continuous supply of glucose to meet energy demands during prolonged exercise bouts (Wasserman et al., 1989).
Resistance Training
Resistance training induces distinct hormonal adaptations to support muscle hypertrophy, strength gains, and metabolic responses:
Insulin: High-intensity resistance exercises stimulate insulin release to enhance amino acid uptake and protein synthesis, crucial for muscle repair, growth, and adaptation to resistance training stimuli (Ivy, 1997).
Glucagon: Glucagon supports glucose mobilization and lipid metabolism during intense resistance exercises, optimizing energy substrate utilization and metabolic efficiency (Richter & Hargreaves, 2013).
Intermittent Exercise
Intermittent exercise patterns, such as interval training or team sports, elicit varied hormonal responses to accommodate alternating periods of high-intensity efforts and recovery:
Insulin: Insulin sensitivity improves with interval training, enhancing glucose uptake and utilization during active intervals while promoting glycogen resynthesis during recovery phases (Richter & Hargreaves, 2013).
Glucagon: Intermittent exercise maintains glucagon secretion to regulate hepatic glucose output and support energy mobilization across varying exercise intensities and metabolic demands (Jensen et al., 1988).
Adaptations in Insulin Sensitivity
Acute Exercise Effects
Acute exercise transiently reduces insulin sensitivity to prioritize glucose delivery to active muscles and support energy production:
Muscle Uptake: Exercise-induced muscle contractions enhance insulin-independent glucose uptake through GLUT4 translocation, promoting immediate energy availability and metabolic efficiency (Richter & Hargreaves, 2013).
Glycogen Depletion: Intense exercise depletes muscle glycogen stores, necessitating post-exercise insulin action to facilitate glycogen resynthesis and promote muscle recovery (Felig & Wahren, 1975).
Chronic Exercise Training
Chronic exercise training enhances insulin sensitivity through various mechanisms, optimizing glucose metabolism and metabolic health:
Mitochondrial Biogenesis: Regular physical activity stimulates mitochondrial adaptation and oxidative capacity in skeletal muscle, promoting insulin-mediated glucose uptake and utilization during exercise and rest (Holloszy & Coyle, 1984).
Muscle Glycogen Storage: Endurance training increases muscle glycogen storage capacity, reducing reliance on hepatic glucose output and enhancing post-exercise glycogen resynthesis facilitated by insulin action (Hawley et al., 2018).
Age-Related Considerations
Aging and sedentary behavior reduce insulin sensitivity, increasing the risk of metabolic disorders and impaired glucose tolerance:
Exercise Intervention: Exercise interventions improve insulin sensitivity in older adults, mitigating age-related declines in metabolic function and supporting healthy aging outcomes (Ivy, 1997).
Combined Training: Combined aerobic and resistance training enhances insulin sensitivity synergistically, optimizing metabolic health and functional capacity across the lifespan (Holloszy & Coyle, 1984).
Clinical Implications and Applications
Diabetes Management
Exercise is integral to diabetes management, improving insulin sensitivity, glucose control, and overall metabolic health:
Type 2 Diabetes: Regular physical activity reduces insulin resistance and enhances glucose uptake, supporting glycemic control and reducing cardiovascular risk factors in individuals with type 2 diabetes (Richter & Hargreaves, 2013).
Exercise Prescription: Tailored exercise prescriptions incorporate aerobic and resistance training modalities to optimize glycemic control, insulin sensitivity, and metabolic outcomes in diabetic populations (Holloszy & Coyle, 1984).
Exercise Guidelines
Optimizing insulin and glucagon responses to exercise requires personalized exercise prescriptions tailored to individual fitness levels, health status, and metabolic goals:
Intensity and Duration: Moderate-intensity aerobic exercise and high-intensity interval training maximize insulin sensitivity and metabolic adaptations, promoting energy expenditure and metabolic flexibility (Hawley et al., 2018).
Resistance Training: Incorporating resistance exercises enhances muscle insulin sensitivity and metabolic rate, supporting muscle growth, and functional capacity across diverse populations (Ivy, 1997).
Hormonal Monitoring
Monitoring insulin and glucagon responses during exercise informs personalized training adjustments and metabolic assessments:
Glucose Management: Continuous glucose monitoring (CGM) systems track blood glucose fluctuations during exercise, optimizing insulin dosing strategies and preventing hypo- or hyperglycemic events (Felig & Wahren, 1975).
Metabolic Assessments: Hormonal profiling and metabolic testing assess individual responses to exercise interventions, guiding therapeutic strategies and optimizing health outcomes in clinical and athletic settings.
Catecholamines Adaptation in Exercise
Catecholamines are essential neurotransmitters and hormones that play a crucial role in regulating various physiological responses during exercise. This text explores the adaptation of catecholamines—epinephrine (adrenaline), norepinephrine (noradrenaline), and dopamine—in response to different types of exercise, their mechanisms of action, and their implications for performance and health.
Overview of Catecholamines
Epinephrine
Epinephrine, also known as adrenaline, is primarily produced by the adrenal glands and plays a pivotal role in the fight-or-flight response. It acts as a hormone and neurotransmitter, facilitating rapid physiological adjustments in response to stressors such as exercise.
Role in Exercise:
Metabolic Effects: Epinephrine stimulates glycogenolysis and lipolysis, releasing glucose and fatty acids into the bloodstream to fuel muscle activity during exercise (Brooks et al., 2005).
Cardiovascular Effects: It increases heart rate and cardiac output, enhancing oxygen delivery to active muscles and supporting aerobic energy production (Fisher et al., 1990).
Respiratory Effects: Epinephrine dilates airways, improving oxygen uptake and respiratory efficiency during exercise (Brooks et al., 2005).
Norepinephrine
Norepinephrine, or noradrenaline, is synthesized in the brain and adrenal glands, acting as both a neurotransmitter in the central nervous system and a hormone in the peripheral nervous system.
Role in Exercise:
Sympathetic Activation: Norepinephrine activates the sympathetic nervous system, enhancing cardiovascular function and promoting vasoconstriction in non-essential organs to redirect blood flow to active muscles (Fisher et al., 1990).
Energy Mobilization: It supports glycogenolysis in skeletal muscle, providing glucose for energy production and maintaining blood glucose levels during prolonged exercise (Brooks et al., 2005).
Mental Alertness: Norepinephrine enhances cognitive function and focus, optimizing mental performance and motor coordination during physical activity (Meeusen & De Meirleir, 1995).
Dopamine
Dopamine functions primarily as a neurotransmitter in the brain, playing a key role in motivation, reward processing, and motor control. Its role in exercise is less understood compared to epinephrine and norepinephrine.
Role in Exercise:
Reward Mechanisms: Dopamine release during exercise contributes to the feelings of pleasure and reward associated with physical activity, motivating continued participation and exercise adherence (Meeusen & De Meirleir, 1995).
Motor Coordination: It modulates motor control and coordination, optimizing movement patterns and skill execution during exercise performance (Harmeier et al., 2020).
Mood Regulation: Dopamine influences mood and emotional responses to exercise, contributing to the psychological benefits of physical activity on mental well-being (Dietrich & McDaniel, 2004).
Hormonal Responses to Different Types of Exercise
Endurance Exercise
Endurance exercise elicits specific hormonal responses that optimize energy metabolism and performance:
Epinephrine: Levels of epinephrine increase proportionally to exercise intensity, supporting metabolic rate, oxygen delivery, and endurance capacity during prolonged aerobic activities (Brooks et al., 2005).
Norepinephrine: Sympathetic activation and norepinephrine release enhance cardiovascular function and energy mobilization, sustaining prolonged muscle activity and aerobic performance (Fisher et al., 1990).
Dopamine: Exercise-induced dopamine release promotes mental alertness and focus, enhancing endurance performance and cognitive resilience during prolonged exertion (Dietrich & McDaniel, 2004).
Resistance Training
Resistance training induces distinct catecholamine responses that optimize muscle contraction, strength gains, and metabolic adaptations:
Epinephrine: Acute resistance exercises increase epinephrine secretion, enhancing muscle contractility, and metabolic rate to support anaerobic energy production and muscle hypertrophy (Fisher et al., 1990).
Norepinephrine: Norepinephrine modulates sympathetic responses, promoting vasoconstriction and blood pressure regulation during intense resistance exercises, optimizing muscle perfusion and strength development (Meeusen & De Meirleir, 1995).
Dopamine: Dopamine release during resistance training enhances motor control and neuromuscular coordination, facilitating optimal movement patterns and muscle recruitment for maximal strength gains (Harmeier et al., 2020).
Interval Training
Interval training elicits varied catecholamine responses, balancing high-intensity efforts with recovery periods to optimize metabolic flexibility and athletic performance:
Epinephrine: Intermittent high-intensity intervals elevate epinephrine levels, promoting rapid energy mobilization, and oxygen delivery to active muscles, enhancing anaerobic capacity and metabolic adaptation (Brooks et al., 2005).
Norepinephrine: Norepinephrine release supports sympathetic activation, regulating blood flow and metabolic responses to alternating exercise intensities, optimizing performance and recovery between intervals (Fisher et al., 1990).
Dopamine: Dopamine modulation during interval training enhances motivation and focus, facilitating mental resilience and adaptive responses to varying exercise intensities and metabolic demands (Dietrich & McDaniel, 2004).
Adaptations in Catecholamine Sensitivity
Acute Exercise Effects
Acute exercise transiently enhances catecholamine sensitivity, optimizing physiological responses to immediate metabolic demands:
Adaptive Signaling: Exercise-induced stress activates catecholamine receptors, enhancing cellular responsiveness to epinephrine and norepinephrine, promoting rapid energy mobilization and metabolic adaptation (Fisher et al., 1990).
Neuromuscular Coordination: Dopamine modulation improves neuromuscular coordination and motor skills, optimizing movement efficiency and performance during dynamic exercise tasks (Harmeier et al., 2020).
Chronic Exercise Training
Chronic exercise training induces long-term adaptations in catecholamine sensitivity, optimizing metabolic efficiency and athletic performance:
Receptor Density: Regular physical activity increases catecholamine receptor density and sensitivity in skeletal muscle and cardiovascular tissues, enhancing sympathetic responsiveness and metabolic regulation during exercise (Meeusen & De Meirleir, 1995).
Endocrine Balance: Balanced catecholamine signaling improves hormonal homeostasis, supporting energy metabolism, cardiovascular health, and stress resilience across diverse exercise modalities (Brooks et al., 2005).
Age-Related Considerations
Age-related declines in catecholamine sensitivity impact exercise performance and metabolic health, requiring tailored interventions to optimize physiological responses:
Exercise Prescription: Age-appropriate exercise prescriptions improve catecholamine sensitivity, enhancing metabolic function, muscle performance, and cardiovascular fitness in older adults (Dietrich & McDaniel, 2004).
Functional Capacity: Regular physical activity mitigates age-related declines in catecholamine responsiveness, preserving functional capacity, and metabolic resilience for healthy aging (Fisher et al., 1990).
Clinical Implications and Applications
Performance Enhancement
Optimizing catecholamine responses through targeted exercise interventions enhances athletic performance and competitive outcomes:
Training Strategies: Tailored exercise programs modulate catecholamine release and sensitivity, improving energy metabolism, endurance capacity, and neuromuscular performance in athletes (Meeusen & De Meirleir, 1995).
Performance Monitoring: Hormonal profiling and metabolic assessments guide personalized training adjustments, optimizing training adaptations and competitive readiness across sports disciplines (Harmeier et al., 2020).
Metabolic Health
Catecholamine adaptations contribute to metabolic health, supporting glucose regulation, lipid metabolism, and cardiovascular function:
Insulin Sensitivity: Enhanced catecholamine signaling improves insulin sensitivity, glucose uptake, and metabolic efficiency, reducing the risk of metabolic disorders and promoting overall health (Brooks et al., 2005).
Weight Management: Exercise-induced catecholamine release facilitates fat oxidation and energy expenditure, supporting weight management and metabolic balance in sedentary and overweight populations (Dietrich & McDaniel, 2004).
Cardiovascular Function
Sympathetic activation and catecholamine release optimize cardiovascular function, enhancing blood flow regulation, and cardiac performance:
Heart Health: Exercise-induced norepinephrine release enhances cardiac contractility and stroke volume, improving cardiovascular efficiency and reducing cardiovascular disease risk (Fisher et al., 1990).
Vascular Adaptation: Catecholamine-mediated vasoconstriction and vasodilation regulate blood pressure and peripheral circulation, supporting vascular health and functional capacity during exercise (Meeusen & De Meirleir, 1995).
Cortisol Adaptation in Exercise
Cortisol, often referred to as the stress hormone, plays a crucial role in the body’s response to physical and psychological stressors, including exercise. This text explores how cortisol levels fluctuate in response to different types of exercise, the mechanisms involved, and the implications for athletic performance and health.
Overview of Cortisol
Role of Cortisol
Cortisol is a steroid hormone produced by the adrenal glands in response to stress. Its primary functions include:
Metabolism: Cortisol increases blood sugar (glucose) levels by promoting gluconeogenesis and inhibiting glucose uptake in cells.
Immune Response: It suppresses the immune system’s inflammatory response.
Stress Response: Cortisol helps regulate the body’s response to stress, including physical exertion during exercise.
Regulation of Cortisol
Cortisol secretion is regulated by the hypothalamic-pituitary-adrenal (HPA) axis. The hypothalamus releases corticotropin-releasing hormone (CRH), which stimulates the pituitary gland to release adrenocorticotropic hormone (ACTH). ACTH then stimulates the adrenal glands to produce cortisol.
Circadian Rhythm
Cortisol levels typically follow a diurnal pattern, peaking in the early morning to help wake up the body and declining throughout the day. Exercise can disrupt this rhythm, leading to acute changes in cortisol secretion.
Hormonal Responses to Different Types of Exercise
Endurance Exercise
Endurance exercise, such as running or cycling, elicits specific cortisol responses:
Moderate Increase: Prolonged aerobic activities moderately elevate cortisol levels due to sustained physical exertion and metabolic demands (Hill et al., 2008).
Duration-Dependent: Longer-duration endurance exercises may lead to higher cortisol levels post-exercise, reflecting prolonged stress on the body’s energy reserves (Viru & Viru, 2001).
Resistance Training
Resistance training induces acute cortisol responses related to muscle damage and metabolic stress:
Immediate Response: High-intensity resistance exercises initially elevate cortisol levels, reflecting acute stress on muscle tissues and metabolic pathways (Kraemer & Ratamess, 2005).
Recovery Phase: Cortisol levels decrease during the recovery phase, as the body repairs and adapts to the mechanical stress placed on muscles (Kraemer & Ratamess, 2005).
Interval Training
Interval training combines high-intensity bursts with periods of rest or low-intensity exercise, influencing cortisol dynamics:
Variable Response: Intense intervals elevate cortisol levels, while recovery periods allow for cortisol reduction and metabolic recovery (Kraemer & Ratamess, 2005).
Metabolic Adaptation: Regular interval training may improve cortisol sensitivity and metabolic efficiency, enhancing performance and recovery (Kraemer & Ratamess, 2005).
Adaptations in Cortisol Sensitivity
Acute Exercise Effects
Acute exercise transiently increases cortisol sensitivity, optimizing metabolic responses to immediate stressors:
Hormonal Signaling: Exercise-induced cortisol release enhances cellular responsiveness to metabolic demands, promoting glucose mobilization and energy production (Hill et al., 2008).
Adaptive Responses: Cortisol modulates immune function and inflammatory responses, supporting tissue repair and adaptation to exercise-induced stress (Viru & Viru, 2001).
Chronic Exercise Training
Regular exercise training induces long-term adaptations in cortisol sensitivity and stress resilience:
Hormonal Balance: Balanced cortisol regulation improves stress management and enhances recovery capacity between exercise sessions (Viru & Viru, 2001).
Neuroendocrine Adaptation: Exercise training optimizes HPA axis function, reducing cortisol reactivity to stressors and promoting hormonal stability (Hill et al., 2008).
Age-Related Considerations
Age-related changes in cortisol sensitivity impact exercise performance and recovery in older adults:
Exercise Prescription: Age-appropriate exercise programs mitigate cortisol dysregulation, enhancing metabolic health and physical function in aging populations (Kraemer & Ratamess, 2005).
Functional Resilience: Regular physical activity supports cortisol balance and adaptive responses to stress, promoting cognitive function and emotional well-being (Kraemer & Ratamess, 2005).
Clinical Implications and Applications
Performance Enhancement
Optimizing cortisol responses through structured exercise interventions enhances athletic performance and recovery outcomes:
Training Strategies: Periodized training programs regulate cortisol fluctuations, improving endurance capacity, and metabolic efficiency in athletes (Kraemer & Ratamess, 2005).
Recovery Protocols: Post-exercise strategies, such as nutrition and rest, mitigate cortisol elevation and promote muscle repair and adaptation (Hill et al., 2008).
Metabolic Health
Cortisol adaptations influence metabolic health, affecting glucose metabolism, lipid profiles, and cardiovascular function:
Insulin Sensitivity: Exercise-induced cortisol release enhances insulin sensitivity and glucose utilization, supporting metabolic homeostasis and reducing diabetes risk (Viru & Viru, 2001).
Weight Management: Cortisol modulation facilitates fat metabolism and energy expenditure, promoting weight management and metabolic balance (Hill et al., 2008).
Stress Management
Physical activity improves cortisol regulation, enhancing stress resilience and mental well-being:
Psychological Benefits: Regular exercise reduces cortisol reactivity to psychological stressors, promoting emotional stability and cognitive function (Viru & Viru, 2001).
Behavioral Interventions: Lifestyle modifications, including exercise and stress management techniques, optimize cortisol responses and promote overall health (Kraemer & Ratamess, 2005).
Growth Hormone Adaptation in Exercise
Growth hormone (GH) is a peptide hormone secreted by the pituitary gland with crucial roles in growth, metabolism, and body composition. This text explores the dynamic interactions between exercise and GH secretion, the mechanisms involved, and the implications for athletic performance and health.
Overview of Growth Hormone
Role of Growth Hormone
GH regulates several physiological processes, including:
Growth: GH promotes tissue growth and development, particularly during childhood and adolescence.
Metabolism: It enhances lipolysis (breakdown of fats) and protein synthesis, supporting energy metabolism and muscle growth.
Repair: GH plays a role in tissue repair and recovery from injuries.
Regulation of Growth Hormone
GH secretion is controlled by complex neuroendocrine mechanisms involving the hypothalamus, pituitary gland, and peripheral feedback loops:
Pulsatile Release: GH is released in a pulsatile manner, with peaks occurring primarily during sleep and in response to exercise, stress, and nutritional intake.
Hypothalamic Regulation: Growth hormone-releasing hormone (GHRH) and somatostatin (GHIH) from the hypothalamus regulate GH release by the pituitary gland.
Physiological Effects
GH exerts direct and indirect effects on various tissues and organs:
Muscle Growth: GH stimulates protein synthesis and muscle cell proliferation, contributing to muscle hypertrophy and strength development.
Bone Health: It enhances bone mineralization and density, promoting skeletal health and integrity.
Metabolic Function: GH enhances glucose uptake and utilization, regulates lipid metabolism, and influences insulin sensitivity.
Hormonal Responses to Different Types of Exercise
Endurance Exercise
Endurance exercise influences GH secretion in a time-dependent manner:
Acute Response: Prolonged aerobic activities initially suppress GH release due to elevated cortisol levels and metabolic demands (Hansen et al., 2008).
Post-Exercise: GH levels increase during recovery, supporting muscle repair and metabolic adaptation to endurance training (Viru & Viru, 2001).
Resistance Training
Resistance exercise elicits robust GH responses related to mechanical stress and muscle damage:
Immediate Response: High-intensity resistance exercises induce acute GH secretion, facilitating muscle protein synthesis and recovery processes (Kraemer & Ratamess, 2005).
Repetitive Stress: Regular resistance training enhances GH pulsatility and sensitivity, promoting muscle hypertrophy and strength gains (Kraemer & Ratamess, 2005).
Interval Training
Interval training combines high-intensity efforts with rest or low-intensity recovery periods, influencing GH dynamics:
Intermittent Peaks: Intense intervals stimulate GH release, while recovery phases maintain anabolic processes and metabolic efficiency (Kraemer & Ratamess, 2005).
Adaptive Benefits: Regular interval training optimizes GH secretion patterns, enhancing muscle adaptation and metabolic flexibility (Hansen et al., 2008).
Adaptations in Growth Hormone Sensitivity
Acute Exercise Effects
Exercise-induced GH release enhances cellular responsiveness to anabolic stimuli:
Hormonal Signaling: GH activates intracellular pathways that promote protein synthesis, muscle repair, and metabolic adaptation to exercise (Hansen et al., 2008).
Adaptive Responses: GH modulates immune function and inflammatory responses, supporting tissue repair and adaptation to exercise-induced stress (Viru & Viru, 2001).
Chronic Exercise Training
Regular exercise training induces long-term adaptations in GH secretion and metabolic regulation:
Hormonal Balance: Balanced GH dynamics improve metabolic efficiency, enhancing energy metabolism and recovery capacity in athletes (Kraemer & Ratamess, 2005).
Neuroendocrine Adaptation: Exercise optimizes GH pulsatility and sensitivity, reducing age-related declines and promoting physiological resilience (Hansen et al., 2008).
Age-Related Considerations
Age-related changes in GH secretion impact exercise performance and recovery in older adults:
Exercise Prescription: Age-appropriate training programs enhance GH secretion, promoting muscle retention, and metabolic health in aging populations (Kraemer & Ratamess, 2005).
Functional Resilience: Regular physical activity supports GH regulation, optimizing anabolic responses and functional independence in older individuals (Viru & Viru, 2001).
Clinical Implications and Applications
Performance Enhancement
Optimizing GH responses through exercise interventions enhances athletic performance and recovery outcomes:
Training Strategies: Periodized exercise programs regulate GH secretion, improving muscle hypertrophy, and endurance capacity in athletes (Kraemer & Ratamess, 2005).
Recovery Protocols: Post-exercise strategies, including nutrition and sleep, optimize GH release and support muscle repair and adaptation (Hansen et al., 2008).
Metabolic Health
GH adaptations influence metabolic health markers, including glucose metabolism and lipid profiles:
Insulin Sensitivity: Exercise-induced GH release enhances insulin sensitivity, promoting glucose uptake, and metabolic homeostasis (Viru & Viru, 2001).
Body Composition: GH facilitates fat metabolism, supporting weight management and lean tissue maintenance in active individuals (Hansen et al., 2008).
Clinical Considerations
Exercise-based interventions modulate GH secretion, offering therapeutic benefits for metabolic disorders and age-related declines:
Endocrine Disorders: Exercise improves GH dynamics in conditions such as growth hormone deficiency and metabolic syndrome, enhancing clinical outcomes and quality of life (Viru & Viru, 2001).
Aging Population: Physical activity mitigates age-related declines in GH secretion, supporting healthy aging and functional independence (Kraemer & Ratamess, 2005).
Testosterone and Estrogen Adaptation in Exercise
Testosterone and estrogen are key hormones that play crucial roles in physiological processes related to exercise adaptation. This text explores their interactions with exercise, the mechanisms involved, and their implications for performance, health, and overall well-being.
Overview of Testosterone
Role of Testosterone
Testosterone is a steroid hormone primarily produced in the testes in males and in smaller amounts in the ovaries and adrenal glands in females. It plays essential roles in:
Muscle Growth: Testosterone stimulates protein synthesis, leading to muscle hypertrophy and strength gains.
Bone Health: It enhances bone mineral density and supports skeletal integrity.
Metabolism: Testosterone influences fat metabolism and insulin sensitivity.
Libido and Mood: It affects sexual function, mood regulation, and cognitive processes.
Regulation of Testosterone
Testosterone secretion is regulated by complex neuroendocrine mechanisms:
Hypothalamic-Pituitary-Gonadal (HPG) Axis: Gonadotropin-releasing hormone (GnRH) from the hypothalamus stimulates luteinizing hormone (LH) and follicle-stimulating hormone (FSH) release from the pituitary gland, which in turn stimulate testosterone production in the testes (in males).
Physiological Effects
Testosterone exerts direct and indirect effects on various tissues and organs:
Muscle Protein Synthesis: Testosterone enhances muscle protein synthesis, contributing to muscle growth and repair.
Bone Mineralization: It supports bone health by increasing calcium retention and bone density.
Metabolic Regulation: Testosterone influences lipid metabolism and insulin sensitivity, promoting energy metabolism and body composition.
Research Insight: Studies have shown that acute resistance exercise can acutely increase testosterone levels in both men and women, with larger responses often seen in men due to their higher baseline levels (Kraemer & Ratamess, 2005).
Estrogen Adaptation in Exercise
Estrogen is a primary sex hormone in females, primarily produced in the ovaries and, to a lesser extent, in adipose tissue and the adrenal glands. It plays essential roles in reproductive health, bone metabolism, cardiovascular function, and overall metabolism. This section explores the impact of exercise on estrogen levels, its physiological effects, and its implications for exercise adaptation.
Estrogen and Exercise
Role of Estrogen
Estrogen has diverse physiological functions:
Bone Health: Estrogen supports bone mineral density and inhibits bone resorption, crucial for skeletal health.
Reproductive Function: It regulates the menstrual cycle, fertility, and secondary sexual characteristics.
Cardiovascular Health: Estrogen has cardioprotective effects, including vasodilation and maintenance of arterial elasticity.
Metabolism: It influences lipid metabolism, glucose homeostasis, and body fat distribution.
Regulation of Estrogen
Estrogen production and activity are regulated by:
Ovarian Function: Estrogen is primarily produced in the ovaries during the reproductive years.
Menstrual Cycle: Estrogen levels fluctuate during the menstrual cycle, peaking during the follicular phase.
Adipose Tissue: Adipose tissue contributes to estrogen production, especially in postmenopausal women.
Physiological Effects
Estrogen affects various tissues and systems:
Bone Density: Estrogen maintains bone mineral density by inhibiting osteoclast activity and promoting osteoblast function.
Cardiovascular System: Estrogen supports cardiovascular health by improving endothelial function and reducing LDL cholesterol.
Metabolic Regulation: Estrogen influences insulin sensitivity and glucose metabolism, contributing to body composition and energy regulation.
Research Insight: Studies suggest that regular physical activity and exercise can modulate estrogen levels, contributing to improved bone health and metabolic function in women (Ennour-Idrissi et al., 2020).
Thyroid Hormone Physiology
The thyroid gland, located in the neck, plays a crucial role in regulating metabolism and influencing various physiological processes throughout the body. Central to its function are the hormones it produces: thyroxine (T4) and triiodothyronine (T3). These hormones are essential for growth, development, and the maintenance of metabolic homeostasis.
Structure and Function of the Thyroid Gland
The thyroid gland consists of two lobes connected by an isthmus, situated anteriorly to the trachea. It synthesizes T4 and T3 primarily from dietary iodine, which is actively transported into the thyroid follicular cells. Within these follicular cells, iodine is incorporated into the amino acid tyrosine to form T4 (containing four iodine atoms) and T3 (containing three iodine atoms).
Thyroxine (T4): T4 is the predominant thyroid hormone secreted by the thyroid gland. It is produced in larger quantities than T3 and serves as a precursor to the more biologically active hormone, T3.
Triiodothyronine (T3): T3 is the more potent thyroid hormone, exerting greater metabolic effects than T4. While most T3 is produced via conversion of T4 in peripheral tissues, a small amount is directly secreted by the thyroid gland.
Regulation of Thyroid Hormone Secretion
Thyroid hormone production is tightly regulated by the hypothalamic-pituitary-thyroid (HPT) axis through a negative feedback mechanism.
Hypothalamus: The hypothalamus releases thyrotropin-releasing hormone (TRH) in response to low circulating levels of thyroid hormones.
Anterior Pituitary Gland: TRH stimulates the anterior pituitary gland to release thyroid-stimulating hormone (TSH), which acts on the thyroid gland to promote the synthesis and secretion of T4 and T3.
Thyroid Gland: TSH binds to receptors on the thyroid follicular cells, increasing iodine uptake, synthesis, and release of T4 and T3 into the bloodstream.
Negative Feedback: Elevated levels of T4 and T3 inhibit TRH and TSH release, thereby reducing thyroid hormone production when levels are adequate.
Mechanism of Action
Thyroid hormones exert their effects by binding to nuclear receptors, which act as transcription factors that regulate gene expression. T3 is more biologically active than T4 and exerts its effects on nearly every tissue in the body, influencing:
Metabolism: T3 increases basal metabolic rate by stimulating oxygen consumption and energy expenditure.
Cardiovascular Function: T3 enhances cardiac output and heart rate, contributing to increased blood flow and tissue perfusion.
Development and Growth: Thyroid hormones are critical for normal growth and development, particularly in the central nervous system and skeletal system.
Thyroid Hormone Adaptation to Exercise
Exercise can modulate thyroid hormone levels in response to metabolic demands and physiological stress:
Acute Response: Intense exercise transiently increases T4 and T3 secretion, enhancing metabolic rate to meet energy demands.
Chronic Adaptations: Regular exercise may lead to long-term changes in thyroid hormone metabolism and sensitivity, potentially lowering resting TSH levels in endurance athletes and altering T3 and T4 conversion rates.
Clinical Relevance
Hypothyroidism: Characterized by insufficient thyroid hormone production, symptoms include fatigue, weight gain, and cold intolerance. Exercise can support management by improving metabolic function and energy levels.
Hyperthyroidism: Excessive thyroid hormone production results in symptoms such as weight loss, heat intolerance, and palpitations. Exercise can help manage symptoms and improve cardiovascular health.
Acute Effects of Exercise on Thyroid Hormones
Increase in Hormone Secretion: During exercise, especially intense aerobic or resistance training, there is a temporary increase in the secretion of thyroid hormones, particularly T4 and to a lesser extent T3. This acute response helps to elevate metabolic rate and energy production to meet the increased demands of the body during physical activity.
Enhanced Peripheral Conversion: Exercise can stimulate the peripheral conversion of T4 to T3. T3 is the more biologically active form of thyroid hormone and plays a crucial role in regulating cellular metabolism and energy expenditure. Increased conversion helps to optimize metabolic efficiency during exercise.
Chronic Adaptations to Exercise
Changes in Thyroid Hormone Metabolism: Regular exercise can lead to long-term adaptations in thyroid hormone metabolism. Endurance athletes, for example, may exhibit lower resting levels of thyroid-stimulating hormone (TSH), which reflects enhanced thyroid hormone sensitivity and efficiency in maintaining metabolic balance.
Impact on Tissue Sensitivity: Exercise training can also influence tissue sensitivity to thyroid hormones. Skeletal muscle, for instance, becomes more responsive to thyroid hormone signaling, which can enhance muscle metabolism and endurance capacity.
Metabolic Efficiency: Improved metabolic efficiency is a hallmark of regular exercise. Enhanced thyroid function supports efficient energy utilization, which contributes to better endurance and overall physical performance.
Clinical Implications
Hypothyroidism: Exercise can be beneficial for individuals with hypothyroidism (low thyroid function) by stimulating thyroid hormone production and improving metabolic rate. However, exercise prescription should be tailored to individual capacity and monitored carefully to avoid exacerbating symptoms.
Hyperthyroidism: Conversely, in individuals with hyperthyroidism (excessive thyroid hormone production), exercise may need to be moderated to prevent overstimulation of the cardiovascular system and exacerbation of symptoms such as palpitations and heat intolerance.
Practical Considerations
Exercise Prescription: Incorporating regular, moderate-intensity aerobic exercise and resistance training can support thyroid health and metabolic function.
Monitoring: Athletes and individuals with thyroid disorders should undergo regular monitoring of thyroid function to optimize performance and manage health effectively.
Adrenal Function and Adaptation in Exercise
The adrenal glands play a pivotal role in the body’s response to stress, including the physical stress imposed by exercise. Comprising the adrenal cortex and adrenal medulla, these glands secrete hormones essential for regulating metabolism, responding to stressors, and maintaining overall homeostasis. Understanding how exercise impacts adrenal function provides insights into optimizing athletic performance and managing stress-related disorders.
Anatomy and Hormonal Output
Adrenal Cortex
The adrenal cortex synthesizes corticosteroids, including glucocorticoids (such as cortisol) and mineralocorticoids (mainly aldosterone). These hormones influence metabolism, electrolyte balance, and stress response. Cortisol, in particular, is crucial for mobilizing energy reserves and modulating inflammation.
Adrenal Medulla
The adrenal medulla produces catecholamines—epinephrine (adrenaline) and norepinephrine (noradrenaline)—which rapidly prepare the body for “fight or flight” responses. These hormones increase heart rate, blood pressure, and glucose availability, enhancing physical readiness during exercise.
Short-Term Effects of Exercise on Adrenal Hormones
Catecholamine Release
During exercise, sympathetic nervous system activation stimulates the adrenal medulla to release epinephrine and norepinephrine. These hormones augment cardiovascular function, dilate airways, and mobilize glucose from glycogen stores, optimizing energy utilization and enhancing physical performance.
Cortisol Response
Exercise induces a transient increase in cortisol secretion from the adrenal cortex. Cortisol helps maintain blood glucose levels by promoting gluconeogenesis (glucose synthesis) and glycogenolysis (glycogen breakdown), ensuring a steady supply of energy for muscles during prolonged activity.
Long-Term Adaptations to Exercise
Enhanced Responsiveness
Regular exercise enhances adrenal responsiveness to stressors, improving the efficiency of catecholamine release and cortisol production. Athletes often exhibit lower resting cortisol levels and quicker recovery post-exercise, reflecting adaptations in adrenal function to support repeated bouts of physical exertion.
Metabolic Adaptations
Endurance training promotes metabolic adaptations in the adrenal cortex, potentially increasing its capacity to produce cortisol. This adaptation supports sustained energy production and aids in buffering against fatigue during prolonged exercise sessions.
Clinical Implications and Considerations
Adrenal Fatigue
Chronic or excessive exercise without adequate recovery can lead to adrenal fatigue—a condition characterized by reduced adrenal hormone production and impaired stress response. Symptoms may include fatigue, mood disturbances, and compromised exercise performance. Balancing training intensity with sufficient rest is crucial for preventing adrenal fatigue in athletes.
Stress-Related Disorders
Exercise serves as a valuable therapeutic tool for managing stress-related disorders like anxiety and depression, partly through its modulation of adrenal hormone secretion. Regular physical activity helps regulate cortisol levels, promote mood stability, and enhance resilience to psychological stressors.
Exercise and Hormonal Health
Exercise influences hormonal health and metabolic regulation, promoting physiological adaptations and overall well-being across the lifespan:
Health Benefits:
Metabolic Control: Regular exercise improves insulin sensitivity, glucose metabolism, and lipid profile, reducing the risk of metabolic disorders such as type 2 diabetes and cardiovascular disease.
Hormonal Balance: Physical activity regulates hormonal secretion and metabolic pathways, supporting endocrine function and reproductive health in athletes and physically active individuals.
Research findings suggest that exercise modulates hormonal responses, optimizing metabolic efficiency and supporting adaptive responses to physiological stressors (Brahimaj et al., 2018). These health benefits highlight the importance of regular physical activity for promoting hormonal health and overall well-being.
Long-Term Adaptations: Chronic exercise enhances hormonal regulation, metabolic flexibility, and resilience to stress, contributing to long-term health outcomes and improved quality of life. Tailored exercise interventions and lifestyle modifications support hormonal balance and optimize health across the lifespan.
Special Populations and Hormonal Adaptations
Certain populations, including athletes, aging adults, and individuals with endocrine disorders, require tailored exercise prescriptions to optimize hormonal adaptations and support health outcomes:
Athletic Performance: Elite athletes benefit from personalized training programs that maximize hormonal responses, enhance performance, and support recovery from training and competition.
Aging and Hormonal Changes: Exercise attenuates age-related hormonal changes, promoting muscle retention, bone density, and metabolic function in older adults. These adaptations optimize physical function and quality of life throughout aging.
Endocrine Disorders: Individuals with diabetes, thyroid disorders, or reproductive hormone imbalances benefit from exercise interventions that improve hormonal regulation, metabolic control, and overall health outcomes.
Research findings underscore the importance of tailored exercise prescriptions and monitoring strategies to optimize hormonal adaptations and support health outcomes in special populations (Hackney & Kallman, 2013). These interventions enhance athletic performance, promote healthy aging, and mitigate risks associated with endocrine disorders.