Carbohydrate
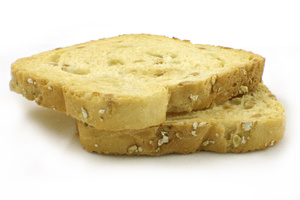
The text delves into the intricate world of carbohydrates, elucidating their composition and multifaceted roles in human physiology. Carbohydrates consist of carbon, hydrogen, and oxygen atoms, with glucose serving as a representative example. While the majority of dietary carbohydrates are derived from plant sources, some are present in animal products, and the liver can produce carbohydrates from specific amino acids and components of fats.
Carbohydrates play pivotal roles in diverse bodily functions, particularly in energy metabolism and exercise performance. Four essential functions of carbohydrates in various tissues are outlined: serving as a metabolic energy fuel for nerve cells and red blood cells, acting as a carbon primer for fat entry into the Krebs cycle, functioning as a metabolic energy fuel for skeletal muscle, and sparing protein from being utilized as an energy source during exercise and intense training.
The primary role of carbohydrates lies in providing metabolic fuel for nerve cells and red blood cells. While nerve tissues can utilize alternative fuel sources in limited quantities, red blood cells exclusively rely on glucose. The brain predominantly uses blood sugar glucose under normal conditions, and maintaining blood glucose levels within narrow limits is crucial for cardiovascular functions, muscle recruitment, and oxygen delivery. Despite their foundational roles in physiological infrastructure, the carbohydrate requirements of nerve cells and red blood cells are often overlooked in the context of exercise metabolism.
Carbohydrates also play a secondary role as an energy fuel for contracting skeletal muscle. The breakdown of carbohydrates produces energy that powers the contractile elements of muscles and other biological processes. Skeletal muscle’s reliance on carbohydrate fuel increases from rest to high-intensity exercise. Additionally, carbohydrate oxidation serves as a crucial step for fat entry into the Krebs cycle, facilitating optimal fat metabolism. Moreover, carbohydrate fuel metabolism aids in sparing adenosine triphosphate (ATP) provision from protein, allowing proteins to focus on their primary roles in tissue structure maintenance, repair, and growth.
The text further explores the diverse types of carbohydrates, emphasizing that not all carbohydrates are equal in form, function, and impact on exercise and sport performance. The fundamental unit of carbohydrates is the monosaccharide, with glucose, fructose, and galactose being the three important dietary monosaccharide sugar molecules in humans. Oligosaccharides, including disaccharides like sucrose, lactose, and maltose, represent carbohydrates composed of 2 to 10 monosaccharides bonded together. The term “complex carbohydrate” or “starch” is commonly used for longer chains or polymers of monosaccharides found in plant-derived foods.
The discussion on monosaccharides emphasizes the central importance of glucose, especially for physically active individuals or athletes undergoing training. While glucose is readily absorbed and available for immediate use by working muscles, fructose and galactose require conversion to glucose in the liver, making them less readily available during exercise.
The concept of oligosaccharides, particularly disaccharides, introduces common sugars like sucrose, lactose, and maltose, each with its unique metabolic characteristics. The text also touches on the prevalence of high-fructose corn syrup in sweetened foods and beverages.
The term “polysaccharide” is then introduced, referring to large carbohydrate substances consisting of 10 to thousands of chemically linked simple sugar molecules. Plant sources contribute starch and fiber, while human and animal tissues store glucose as the polysaccharide glycogen. Starch, the storage form of glucose in plants, exists in two forms: amylose and amylopectin. The relative proportions of these forms in a plant food influence its digestibility, with amylopectin-rich starches digesting well and being absorbed readily in the small intestine.
The comprehensive exploration of carbohydrates extends beyond their chemical structure, shedding light on their intricate functions, diverse types, and implications for exercise and sport performance. Athletes are encouraged to understand the distinctions among carbohydrate types, recognizing their roles in replenishing muscle glycogen, maintaining blood glucose levels during competition, and promoting overall health, particularly cardiovascular health.
The text provides a detailed exploration of fiber and glycogen, shedding light on their classifications, functions, and significance for human health and athletic performance.
Fiber, classified as a structural, nonstarch polysaccharide, is categorized into three types: dietary fiber, functional fiber, and total fiber. Dietary fiber encompasses nondigestible carbohydrates and lignin found in plants, including digestion-resistant starch. Functional fiber consists of isolated, nondigestible carbohydrates with beneficial effects in humans, contributing to health-enhancing outcomes. Total fiber is the sum of dietary fiber and functional fiber. Fibers exhibit diverse physical and chemical characteristics, with cellulose, hemicellulose, and pectin found in various plant structures. Water-insoluble fibers, like cellulose and hemicellulose, aid gastrointestinal function by scraping intestinal wall cells, while water-soluble fibers, including psyllium seed husk and beta-glucan, shorten food residue transit time through the digestive tract. The text notes that the typical American diet falls short of the recommended daily fiber intake, emphasizing the potential health benefits associated with adequate fiber consumption.
The discussion on fiber extends to its linkages with chronic diseases, with high fiber intake, particularly from whole-grain cereal fibers, associated with a lower risk of heart disease, peripheral artery disease, hyperlipidemia, obesity, diabetes, and gastrointestinal cancers. While fiber itself does not directly impact athletic performance, its consumption supports overall health and disease prevention.
Shifting focus to glycogen, the text defines it as a large, branched polymer of glucose units serving as the body’s carbohydrate storage form. Glycogen, irregularly shaped and highly branched, consists of hundreds to thousands of glucose units forming dense granules. Liver and skeletal muscles are the major sites of glycogen storage, with skeletal muscles storing more total glycogen due to their greater mass. Glycogen’s presence significantly increases the immediately available carbohydrate between meals and during muscular contraction. The concentration of glycogen is higher in the liver, playing a crucial role in blood glucose homeostasis regulation by the hormone insulin. Insulin promotes skeletal muscle blood flow, stimulates glucose uptake, glycolysis, and glycogen synthesis in skeletal muscles. Glycolysis, the breakdown of carbohydrates (glucose) to produce ATP, is a key process in this context.
The importance of maximizing glycogen stores is highlighted, particularly for aerobic endurance athletes and those engaged in high-intensity training. Insulin, a pancreatic hormone, is introduced as a key regulator of blood glucose levels, responding to elevated blood glucose and amino acid concentrations by increasing tissue uptake of both.
In summary, the text provides a comprehensive understanding of fiber and glycogen, elucidating their classifications, functions, and roles in promoting health and supporting athletic performance.
The text delves into the concept of Glycemic Index (GI), elucidating its definition and its role in determining the rate at which glucose levels rise in the blood after consuming 50 g of a specific food. The GI of a food is influenced by the availability of ingested carbohydrates to enzymes in the small intestine, dictated by factors such as gastric emptying and the physical presence of sugar or starch for intestinal enzymes. It highlights that slow absorption rates and a low GI are associated with certain foods like brown rice, whole-grain pasta, and multigrain breads, while high-GI foods, including refined table sugar and white rice, lead to a rapid but transient increase in blood glucose and insulin production. The text notes that cooking can alter the glycemic index, making it imperative to consider such factors when predicting the glycemic indexes of different carbohydrate sources, be they liquid or solid.
The discussion shifts to the importance of carbohydrates in exercise preparation, performance, and recovery, emphasizing increased carbohydrate requirements for athletes engaged in repetitive training. Athletes can strategically use both high- and lower-glycemic carbohydrate foods to optimize performance in various contexts. High-glycemic carbohydrate sources are crucial for maintaining blood glucose levels during prolonged aerobic endurance exercise and for rapid recovery of muscle glycogen after exercise. On the other hand, slower-absorbed, unrefined, complex carbohydrates are beneficial for optimizing muscle carbohydrate storage between exercise bouts, preventing drastic fluctuations in blood glucose levels during prolonged recovery.
The subsequent section delves into the regulation of carbohydrates in the body, encompassing the maintenance of blood glucose, glycogen synthesis, and glycogen degradation. It emphasizes the essential yet limited role of carbohydrates as a fuel source in the body, with the liver, pancreas, and other organs working to keep blood glucose levels within a narrow range during rest. The balance of hormones like glucagon and insulin plays a crucial role in regulating blood glucose levels. Glucagon is released when blood sugar falls below normal, mobilizing glucose precursors for gluconeogenesis in the liver, while insulin is released when blood glucose levels rise after a meal, promoting glucose removal from the blood, storage as glycogen, and inhibiting glycogenolysis and gluconeogenesis.
The text elaborates on glycogen synthesis, detailing the intracellular modifications of glucose to form UDP-glucose, which is then added to the growing glycogen molecule by the enzyme glycogen synthase. Glycogen is portrayed not as a simple string of repeated glucose compounds but as a highly branched polymer, facilitating rapid synthesis and degradation crucial for providing glucose during high-intensity exercise. Additionally, the process of glycogen breakdown during exercise is explored, aiming to release glucose-1-phosphate compounds for quick ATP production, essential for fueling skeletal muscle contraction. The role of glucose-6-phosphate in cellular glucose metabolism, connecting glucose storage and oxidation, is emphasized in this context.
In essence, the text provides a thorough exploration of the Glycemic Index, its implications for carbohydrate sources, and the intricate processes involved in carbohydrate regulation, synthesis, and breakdown within the body.
The text delves into the intricacies of glycolysis, emphasizing its crucial role in rapidly generating ATP for energy production during exercise, intense training, and sport performance. Occurring in the cytoplasm of muscle fibers, glycolysis involves the breakdown of carbohydrates, specifically glucose, to produce ATP. It is depicted as a series of 10 enzymatically controlled chemical reactions, initiating with a six-carbon glucose molecule and culminating in the production of two three-carbon pyruvate molecules. The quick ATP production resulting from glycolysis is vital for muscle contraction during high-intensity training or exercise.
The fate of pyruvate, the end product of glycolysis, is explored, presenting two possibilities: conversion to lactate or entry into the mitochondria. The subsequent section details the production of lactic acid, which occurs through anaerobic glycolysis when pyruvate is converted to lactic acid. The text underscores the significance of adequate carbohydrate intake in the diet to fuel intense exercise, as glycolysis predominantly relies on the oxidation of glucose for ATP generation. The mitochondria, responsible for ATP production with oxygen, play a pivotal role in further metabolizing pyruvate through the Krebs cycle.
The discussion then shifts to lactic acid production and clearance, elucidating how pyruvate, when converted to lactic acid, can rapidly ionize, releasing hydrogen ions and lowering sarcoplasmic pH. The ionized molecule becomes lactate, and the text emphasizes the importance of buffering lactic acid inside the cell or expelling it for extracellular buffering. Plasma hemoglobin and bicarbonate are highlighted as prominent extracellular buffers. The rise in blood levels of lactate during higher-intensity exercise is detailed, with lactic acid production aiding anaerobic ATP production from carbohydrates for a brief period. The “burning” sensation during high-intensity muscle contraction is attributed to the lower pH irritating free nerve endings.
Fatigue is linked to the accumulation of lactic acid, causing a drop in pH in working muscles. Sustained, higher-intensity contractions can deplete muscle glycogen, precipitating muscle fatigue as the anaerobic system’s ability to sustain rapid ATP resynthesis is compromised. While lactate accumulation correlates with fatigue, the text notes that there are no nutritional practices to decrease lactate production during intense exercise. Conditioning is highlighted as a factor enabling athletes to work at higher intensities with lower associated lactate levels. Optimal carbohydrate intake is emphasized to facilitate high-intensity training, promoting adaptations that favor energy production from oxidative sources of energy.
The text concludes by emphasizing that carbohydrate availability in the body controls its use for energy. Blood glucose concentration provides feedback regulation of the liver’s glucose output, with increased blood glucose inhibiting hepatic glucose release during exercise. Carbohydrate availability may limit fat metabolism by reducing fatty acid mobilization and oxidation in the cell, aligning with the metabolic demands of high-intensity exercise.
The text explores the pivotal role of carbohydrates in various types of exercise training and sport performance, encompassing both aerobic and anaerobic activities. The discussion begins by acknowledging the diverse nature of athletes, some primarily engaging in aerobic exercises and others in anaerobic activities, and highlights the universal performance-enhancing benefits of resistance exercise. The subsequent exploration encompasses the significance of carbohydrates in strength training, as well as considerations related to both aerobic and anaerobic exercise and performance.
In the realm of aerobic exercise, the liver’s role in glucose production to maintain blood glucose concentration is elucidated. Blood glucose contributes significantly to the energy fuel required by exercising muscles, with the additional support of stored muscle glycogen. However, prolonged and intense aerobic exercise leads to a decline in blood glucose concentration due to liver glycogen depletion, while active skeletal muscles continue to utilize available blood glucose. The text emphasizes the potential concern of glycogen depletion, particularly when starting exercise sessions with suboptimal glycogen levels. The negative impact of carbohydrate-deficient diets on performance, despite potential fat loss benefits, is highlighted. The decline in muscle glycogen levels late in exercise is linked to increased reliance on blood glucose, leading to hypoglycemia, impairing exercise performance, and contributing to central nervous system fatigue.
Fatigue during prolonged aerobic exercise is attributed primarily to depleted carbohydrate stores in exercising muscles, even with an ample oxygen supply and abundant stored energy from fat. The phenomenon, commonly known as “hitting the wall,” manifests as weakness, dizziness, decreased motivation, and a decline in muscle glycogen, ultimately leading to exercise termination. Optimizing preexercise muscle glycogen stores is emphasized, showcasing its positive impact on time to exhaustion and aerobic endurance performance. The text also introduces carbohydrate consumption strategies during exercise lasting 45 minutes or more to maintain blood glucose levels and improve endurance capacity. The postexercise period is underscored as essential for replenishing muscle glycogen, preparing for upcoming challenges.
Transitioning to anaerobic exercise, the text highlights skeletal muscle glycogen as a readily available energy source, with its utilization highly dependent on exercise intensity. As exercise intensity increases, muscle glycogen becomes a crucial energy substrate, especially above the anaerobic threshold or during high-intensity exercise. Depletion of muscle glycogen to a certain threshold leads to increased reliance on blood glucose uptake. The significance of muscle carbohydrate stores is particularly relevant during prolonged and intermittent high-intensity exercise bouts. The importance of daily carbohydrate consumption for anaerobic athletes is emphasized, aligning with the optimization of preexercise muscle glycogen levels and rapid replenishment post-exercise.
In summary, the text underscores the indispensable role of carbohydrates in supporting various types of exercise, whether aerobic or anaerobic, and highlights the nuanced considerations for optimal performance in each scenario. The discussion emphasizes the interplay between carbohydrate intake, glycogen levels, and exercise performance, providing insights into strategies for athletes in both domains.
The text delves into the role of carbohydrates in the context of strength training, encompassing aspects of muscular strength, endurance, and power. Strength performance, characterized by repetitive bouts of high-intensity work with short rest intervals, relies significantly on carbohydrates as the primary fuel source. Similar to anaerobic exercise, the intensity of the strength training session dictates the recruitment of fast-twitch muscle fibers, particularly Type IIx fibers, which fatigue quickly as their glycogen content is utilized.
During high-intensity resistance exercise, the recruitment of fast-twitch fibers is pronounced, especially during eccentric and high-speed contractions. Notably, research indicates that even moderate and lower-intensity muscle contractions engage faster-twitch fiber types. This insight suggests that the fatigue associated with muscular endurance exercises could be influenced by the initial glycogen content and rate of depletion in recruited fast-twitch fibers. Given that strength and power athletes engage in intense training multiple times a week, maintaining adequate carbohydrate intake becomes crucial to prevent gradual glycogen depletion in trained muscles over time.
The cumulative glycogen use in resistance-trained muscles throughout a workout, including warm-up, stretching, and cool-down sessions, raises the proposition that higher carbohydrate consumption could enhance muscle performance in these activities. However, research studies present varied results regarding specific nutritional carbohydrate practices and acute strength training performance. Despite the lack of consensus on high-carbohydrate diet recommendations or carbohydrate ingestion before weightlifting, the text emphasizes the undeniable role of skeletal muscle carbohydrate sources in facilitating overall resistance exercise performance. This becomes especially critical during weight training sessions involving multiple muscles or muscle groups worked to the point of fatigue, potentially leading to glycogen depletion and a prolonged postexercise period of energy-consuming muscle recovery.
Optimal carbohydrate ingestion is deemed imperative for the overall outcome of the training regimen, potentially affecting increased strength and power. Additionally, the text underscores the impact of carbohydrate ingestion on insulin levels, particularly the significant increase in endogenous insulin secretion with carbohydrate intake, especially high-glycemic types. Insulin, acting as a potent anabolic hormone in previously exercised muscles, plays a role in promoting protein synthesis, decreasing protein breakdown, stimulating glucose uptake, and enhancing glycogen storage. These effects, in conjunction with recommendations for liquid carbohydrate ingestion before, during, and after exercise, aim to expedite recovery and promote gains in lean body mass.