Nutrition for Strength Adaptations
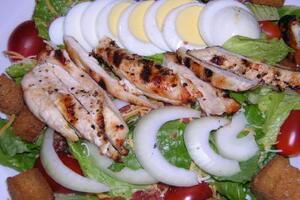
The text delves into the significance of muscle mass, constituting approximately 45% of body weight and serving as the most abundant tissue. Beyond its role in strength, muscle mass significantly influences basal metabolic rate, positioning it as a crucial element in the battle against metabolic diseases. The dynamic nature of muscle mass throughout life is highlighted, showcasing an increase in strength and mass until the second decade, followed by a decline from the fourth decade onward. This life-long variation underscores the centrality of muscle mass to human longevity, with those in the strongest third of the population being 2.5 times more likely to reach their 100th birthday.
The plasticity of muscle as a tissue is emphasized, responding rapidly to changes in loading and nutritional conditions. Exercise of sufficient frequency, intensity, and duration promotes muscle mass increase, while decreased loading leads to rapid muscle mass loss. The text directs attention to the role of nutrition in tandem with training in regulating muscle size and strength. Muscle strength, determined by factors such as cross-sectional area, neural control, and force transfer ability, is explored. Cross-sectional area, in particular, is influenced by the balance between muscle protein synthesis (MPS) and muscle protein breakdown (MPB), with muscle hypertrophy occurring when MPS rates exceed those of MPB.
The narrative then shifts focus to nutritional interventions, starting with protein consumption. Protein emerges as a key factor with robust evidence supporting its role in enhancing muscle mass increase during training. A meta-analysis of 22 randomized clinical trials underscores the significant impact of protein supplementation on both muscle mass and strength. The importance of essential amino acids (EAAs), particularly leucine, is highlighted in promoting muscle protein balance and stimulating MPS. Leucine-rich and readily digestible proteins are identified as optimal triggers for MPS, with ground meat and whey protein exemplified as effective sources.
The text introduces the concept of protein quantity, emphasizing a dose-dependent relationship between protein intake and MPS. The optimal protein dose for increasing muscle mass in young men is identified as 0.25 g/kg body weight. However, populations such as older individuals or those in a caloric deficit may require higher doses (∼0.4 g/kg body weight) within a single serving. The molecular mechanism underlying the protein synthetic response to feeding involves the activation of mTORC1, a critical signaling molecule.
While the role of hormones like insulin and IGF-1 in response to feeding suggests a potential benefit of adding carbohydrates to post-resistance exercise meals, the text dismisses this notion. It explains that resistance exercise can independently move the inhibitor TSC2 away from Rheb, thereby activating mTORC1 without relying on hormonal signals. This prolonged sensitivity to amino acids after exercise, especially leucine-rich protein, enhances the MPS response for up to 24 hours.
The section concludes by addressing branched-chain amino acids (BCAAs) and their role in muscle size and strength. While BCAAs, particularly leucine, are recognized for stimulating MPS after exercise, conflicting reports exist regarding their impact on muscle size and strength with training. The text suggests that while BCAAs can increase MPS, they may not be as effective as complete proteins, which provide all essential amino acid building blocks necessary to maximize MPS.
The text explores the role of beta-hydroxy beta-methylbutyric acid (HMB) in the context of muscle protein balance, similar to branched-chain amino acids (BCAAs). HMB, a metabolite of leucine, initially garnered attention for its potential to increase muscle mass and strength. Early reports suggested positive effects with daily HMB supplementation ranging from 1.5 to 3 g. However, conflicting findings emerged, with some studies failing to show benefits in either strength or mass. Notably, discrepancies in study duration and measurement methods, such as underwater weighing versus dual-energy X-ray absorptiometry, may contribute to the conflicting results. Recent studies by Wilson and colleagues have reignited interest in HMB, proposing that 3 g of the free acid form of HMB per day for 12 weeks can lead to substantial gains in strength, power, and muscle mass. However, these findings have faced scrutiny within the scientific community, and the true efficacy of HMB likely lies between divergent study outcomes.
The text delves into the potential mechanisms through which HMB may exert its effects. HMB is suggested to activate mTORC1 signaling in both human and porcine muscle. The precise mechanism remains unclear, but hypotheses include product inhibition of leucine breakdown, leading to increased intramuscular leucine that activates mTORC1. Comparisons between HMB and high leucine-containing proteins reveal that the latter is more effective at activating mTORC1 and increasing muscle protein synthesis (MPS). Despite equivocal evidence regarding HMB’s impact on MPS and strength gains, its ability to prevent muscle mass and strength loss, especially during periods of inactivity, appears more consistent. Studies indicate that HMB supplementation, as well as high leucine intake, can mitigate muscle wasting during bed rest, with leucine-rich protein showing superiority in maintaining muscle mass and strength.
The text then transitions to the discussion of creatine, a widely used ergogenic aid and popular supplement among athletes seeking to enhance strength performance. Creatine’s fame grew after athletes like Linford Christie and Sally Gunnell credited their success at the 1992 Barcelona Olympic Games to creatine supplementation. The primary function of creatine is to maintain adenosine triphosphate (ATP) levels during exercise, particularly in short-duration, maximal intensity activities such as strength training or sprinting. The text explains the ATP replenishing role of the phosphocreatine (PCr) system, highlighting how increasing intramuscular creatine levels through supplementation can augment the capacity of this system and improve performance.
Daily creatine turnover is approximately 2 g, necessitating a dietary intake of 1–3 g to maintain adequate skeletal muscle creatine levels. Vegetarian athletes, relying on lower dietary creatine sources, often exhibit lower intramuscular creatine stores. Creatine supplementation typically involves a loading phase followed by a maintenance phase, with doses tailored to body weight. Two meta-analyses confirm the effectiveness of creatine supplementation in augmenting lower and upper limb strength performance. Studies show positive effects on squat and leg press performance, bench press performance, and pectoral muscle strength. Notably, vegetarians, starting with lower baseline creatine levels, experience robust responses to creatine supplementation.
Gender differences in response to creatine supplementation are discussed, with men generally exhibiting greater gains in muscle mass and strength compared to women. The text attributes this difference to potential higher rates of anticatabolic processes in men. While the exact mechanistic details of creatine’s impact on muscle size and strength remain elusive, evidence suggests that creatine increases muscle mass and strength. Studies also indicate that creatine supplementation, particularly in vegetarians and young athletes, is generally safe and well-tolerated.
The text explores the role of β-alanine in the context of strength training, emphasizing the importance of intense, short-duration exercises. During such activities, muscle contractions primarily rely on ATP derived from glycolysis, leading to the accumulation of lactate and protons, causing acute acidification. To counteract this, skeletal muscles contain the dipeptide carnosine, an intracellular buffering agent that mitigates muscle acidosis and delays fatigue. Carnosine, synthesized within muscles, is particularly abundant in type II fibers. However, its levels vary based on factors like fiber type, gender, age, and diet.
While carnosine’s role in pH buffering is well-established, other physiological roles have been proposed, such as contributing to excitation–contraction coupling, particularly in calcium handling. Carnosine synthesis is limited by β-alanine availability, and vegetarians may have lower carnosine levels. Dietary sources of β-alanine include poultry, red meat, and fish. The total amount of ingested β-alanine, rather than baseline carnosine levels, determines the change in muscle carnosine levels.
The text discusses the inconclusive evidence regarding the impact of exercise training on muscle carnosine levels. Short-term interventions generally show no effect, but long-term training may alter levels by affecting fiber-type composition. β-alanine supplementation protocols recommended by the International Society of Sports Nutrition involve daily ingestion for at least 2-4 weeks to achieve significant increases in muscle carnosine levels. Large boluses of β-alanine can induce side effects, so controlled, sustained-release methods or small doses throughout the day are advised. Co-ingestion with a mixed meal enhances its effectiveness. β-alanine loading can be followed by maintenance with lower daily doses.
Regarding strength performance, the text highlights that β-alanine’s effects are not extensively studied. Meta-analyses suggest efficacy in exercises lasting 1-4 minutes but less impact in shorter or longer durations. Studies on strength performance outcomes are equivocal, with some indicating increased isometric endurance and improved squat exercise performance. The impact on isokinetic force production, whole-body strength, and muscular endurance is inconclusive.
β-alanine supplementation’s consistent benefits lie in attenuating fatigue during repeated dynamic contractions and enhancing training volumes. This can lead to improved training quality and capacity, potentially resulting in strength gains. Despite limited and somewhat mixed data on strength outcomes, β-alanine’s positive effects on fatigue resistance and training volume make it a promising ergogenic aid. The text acknowledges the nascent state of research on β-alanine’s role in strength performance but suggests its potential application across trained and untrained athletes of various genders and ages.
The discussion then shifts to Ω3 fatty acids, specifically eicosapentaenoic acid (EPA) and docosahexaenoic acid (DHA). These essential fatty acids, derived from cold-water fatty fish and flaxseed oil, are proposed to enhance muscle remodeling and repair through increased muscle protein synthesis (MPS). The text notes that assertions about Ω3 PUFAs’ impact on MPS are based on limited studies, often involving elderly individuals or animals.
Studies on young and older adults indicate that daily consumption of EPA and DHA increases MPS and the phosphorylation of mTORC1 target proteins. The proposed mechanism involves the incorporation of Ω3 PUFAs into muscle phospholipid membranes, promoting the association of mTOR with activators after resistance exercise and feeding. Notably, the positive effect is attributed to EPA rather than DHA.
The impact of Ω3 PUFA supplementation on MPS appears to be influenced by factors such as age, gender, and training status. While studies on young men show no advantage in MPS when a sufficient protein dose is ingested, older women demonstrate improvements in strength, muscle quality, and functional measures. The text suggests that Ω3 PUFAs may decrease contraction-induced injury, leading to enhanced adaptation in cases where muscle damage limits performance.