Sports Biomechanic
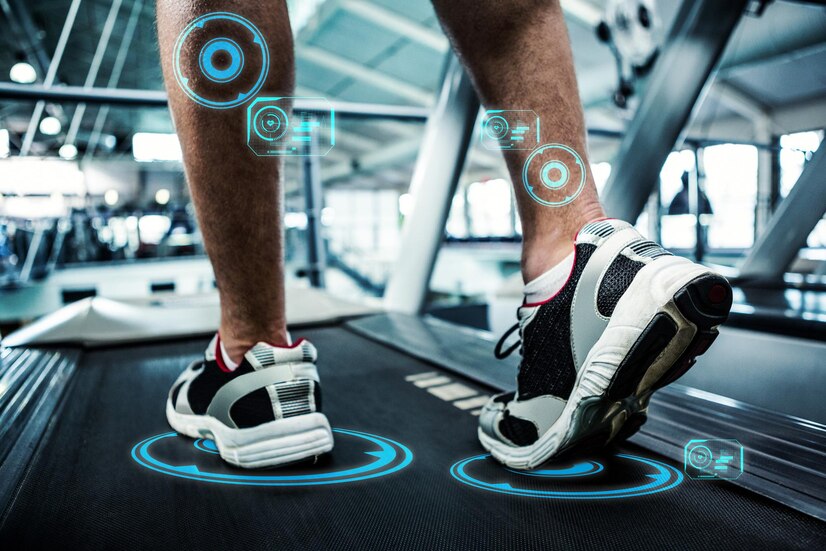
The Motivation for Studying Biomechanics
In this section, the text explores the motivations and benefits of studying biomechanics. It addresses several fundamental questions that readers, typically undergraduate students majoring in kinesiology, physical education, exercise science, or other human movement sciences, might have regarding the value of learning biomechanics. The text begins by raising key questions: What motivates someone to study biomechanics? What practical gains can be derived from acquiring knowledge in biomechanics? How does understanding biomechanics contribute to one’s future pursuits? Is the time invested in learning biomechanics worthwhile? These questions are posed to encourage readers to reflect on the importance of studying this field.
It acknowledges that many readers may find themselves studying biomechanics as part of a mandatory course, primarily for academic credit to graduate. For these readers, it might be challenging to understand the broader implications and benefits of biomechanics due to limited prior exposure to the subject. To address this, the text offers reasons for studying biomechanics, intending to motivate students and provide context for their learning journey. The text highlights that most readers, aspiring to become physical education teachers, coaches, or other physical activity specialists, are likely involved in various sports and fitness activities. It presents a scenario where students or athletes ask questions about the “why” of certain techniques or skills. It underscores the role of biomechanics in answering these questions, explaining not only what techniques to teach or coach but also why those techniques are the most effective. By studying biomechanics, readers can better evaluate unfamiliar sport skills and improve their assessment of familiar ones.
Furthermore, the text emphasizes the relevance of biomechanics for students in athletic training, physical therapy, and sports medicine. It explains that biomechanics can help diagnose the causes of injuries and provide the mechanical foundation for techniques like taping, braces, and orthotic devices. Additionally, a solid understanding of biomechanics can guide therapists in devising rehabilitation plans and assist exercise specialists in identifying exercises that may be risky for certain individuals.
Defining Biomechanics:
In this section, the text aims to provide readers with a comprehensive understanding of the concept of biomechanics. It begins by asking fundamental questions about what biomechanics is and how it has been understood and utilized. The primary objective is to offer a clear definition of biomechanics and highlight the diversity of its applications.
The text acknowledges that many readers might associate biomechanics with evaluating the optimal techniques used by athletes in various sports. While biomechanics indeed plays a significant role in technique analysis, it emphasizes that biomechanics encompasses a more extensive scope. To gain insight into the definition of biomechanics, the text looks to the library for clues. It mentions several journals and publications with “biomechanics” in their titles, such as the Journal of Biomechanics, the Journal of Biomechanical Engineering, and others. By examining the articles featured in these journals, the text reveals that while some focus on sport biomechanics, others cover a wide range of seemingly unrelated topics. These topics include studies on the functioning of the heart’s mitral valve, human cornea, pulmonary airflow, and even the biomechanics of fruits and vegetables. This demonstrates the far-reaching applications of biomechanics, which extend beyond human activities and even encompass non-human biological systems.
The text further dissects the word “biomechanics” to uncover its inherent meaning. It deconstructs the term into two parts: “bio-” and “mechanics.” The prefix “bio-” implies a connection to living or biological systems, while “mechanics” suggests an analysis of forces and their consequences. This analysis leads to the conclusion that biomechanics is the study of forces and their effects on living systems. To reinforce this definition, the text references Herbert Hatze’s 1974 definition of biomechanics as “the study of the structure and function of biological systems by means of the methods of mechanics.” This definition highlights the extensive scope of biomechanics, encompassing the study of both plants and animals’ structure and function.
The Role of Biomechanics in Sport Performance Improvement
This section explores the specific goals and applications of biomechanics in the context of sport and exercise. The text differentiates between the broader field of biomechanics, which encompasses all living things, and sport and exercise biomechanics, which exclusively focuses on humans involved in exercise and sport.
The primary objective of sport and exercise biomechanics is defined as performance improvement in exercise and sport. This goal is further emphasized by the secondary objective of injury prevention and rehabilitation, which is closely intertwined with performance enhancement. The text asserts that maintaining an athlete’s physical well-being is vital because an uninjured athlete is more likely to perform better. Biomechanics work toward achieving these goals through various means, with technique improvement being one of the central methods. The text introduces two primary scenarios where biomechanics plays a role in enhancing performance through technique improvement:
- Teachers and Coaches: Teachers and coaches employ qualitative biomechanical analysis methods in their instructional roles. They use their knowledge of mechanics to identify areas where a student or athlete can enhance their performance. These observations often lead to suggestions on how to alter technique for better results, aligning with the coach’s expertise in biomechanical principles.
- Biomechanics Researchers: Biomechanics researchers use quantitative biomechanical analysis methods to investigate new and more effective techniques for sport skills. Their findings can revolutionize training and coaching by introducing novel approaches. The text acknowledges that while groundbreaking, revolutionary techniques are rare, biomechanics research often leads to small refinements in technique.
To illustrate these two scenarios, the text provides examples from various sports, including swimming, javelin throwing, high jumping, and cross-country skiing. Notably, some of these techniques were developed without significant input from biomechanics research, suggesting the ingenuity and skill of coaches, athletes, and teachers. However, it also poses the idea that a better understanding of biomechanics may have expedited the development of these improved techniques.
Biomechanics’ Impact on Equipment and Performance Improvement
This section discusses the role of biomechanics in enhancing sports performance through equipment improvement. It questions how changes in equipment design can affect an athlete’s performance and examines several sports where such changes have significantly impacted performance.
The text starts by highlighting the essential role that shoes and apparel play in various sports, indicating that these pieces of equipment can directly influence performance or contribute to injury prevention. It prompts the reader to consider examples of sports where innovations in apparel or shoes have led to performance improvements, such as swimming, ski jumping, and speed skating. Using swimming as an illustrative example, the text delves into the evolution of swimsuit design and how it has influenced performances over the years. It narrates how the introduction of Speedo’s LZR Racer swimsuit in 2008, designed with insights from biomechanics, brought about a significant surge in world records and gold medals at the Beijing Olympic Games.
The text then extends its focus to other sports equipment and implements, pondering how changes in equipment design have affected sports such as cycling, skiing, tennis, golf, pole vaulting, and javelin throwing. It introduces the story of the Held brothers, who, despite not being biomechanists, applied their knowledge of mechanics to design a more aerodynamic javelin. Their innovation led to remarkable improvements in javelin throwing distances and the setting of new world records.
It further examines how regulations and rules in many sports aim to maintain the challenge by controlling equipment design. For example, governing bodies in sports like golf, tennis, cycling, and baseball regulate equipment designs to ensure fair play. However, the text underscores that recent equipment innovations have sometimes resulted in significant changes in performance, despite regulatory efforts. The text offers examples of how modern equipment, such as the “klap” skate, contributed to a series of world records in speed skating at the 1998 Winter Olympics. It also revisits the impact of the Speedo LZR swimsuit on swimming events during the 2008 Beijing Olympics, underscoring that changes in equipment can lead to groundbreaking performances.
Biomechanics’ Role in Training Improvement for Enhanced Sports Performance
This section explores how biomechanics can significantly contribute to improving sports performance by enhancing training methods. It suggests that biomechanical analyses of athletes’ techniques can assist coaches and teachers in identifying the specific training requirements necessary for performance improvement. Several examples are provided to illustrate how biomechanics can impact training and lead to better performances.
The text begins by posing the question of how biomechanics can play a role in refining training methods to boost athletic performance. It explains that biomechanics offers insights into the technique deficiencies of athletes, which, in turn, can guide coaches and teachers in tailoring training programs to address these limitations. These deficiencies may relate to muscle strength, endurance, speed of movement, or particular aspects of technique.
Using gymnastics as an example, the text illustrates that biomechanics can pinpoint the need for strength in specific muscle groups. It mentions the importance of strong adductor muscles of the shoulder for gymnasts attempting maneuvers like the iron cross. While some limitations in sports techniques are apparent, such as in gymnastics, others may not be as obvious, as highlighted in pole vaulting, especially among high school-age athletes. The text points out that many novice pole vaulters struggle to achieve an inverted position due to deficiencies in shoulder extensor strength. Biomechanical analysis can unveil this issue and inform the development of targeted training programs to enhance the vaulter’s shoulder extensor muscles, ultimately improving their technique.
The text concludes with a final example in cross-country skiing from the late 1970s. It describes how a biomechanical analysis of an international competition provided insights into the American skiers’ strengths and weaknesses on different sections of the course. The analysis indicated that the American skiers excelled on flat and downhill sections but lagged behind on uphills. Armed with this information, coaches adjusted their training programs, dedicating more time to uphill training and refining the skiers’ uphill techniques to improve their overall performance.
The Role of Biomechanics in Injury Prevention and Rehabilitation in Sports and Exercise
This section discusses the significant role of biomechanics in injury prevention and rehabilitation within the realm of sports and exercise. It suggests that for some, injury prevention and rehabilitation should be the primary focus of sport and exercise biomechanics. The field of biomechanics is shown to be highly valuable for sports medicine professionals, enabling them to identify the forces responsible for injuries, create strategies to prevent recurring or initial injuries, and develop rehabilitation exercises. Biomechanics provides the foundation for making adjustments in technique, equipment design, and training to either prevent or aid in the rehabilitation of injuries.
The text provides specific examples to illustrate the ways in which biomechanics can contribute to injury prevention and rehabilitation. One such example pertains to the field of gymnastics. Research funded by the United States Olympic Committee and the United States Gymnastics Association focuses on understanding the impact forces experienced by gymnasts when landing from stunts and the strategies they employ to minimize these forces. Gymnasts are awarded higher scores for ‘sticking’ their landings, yet these landings can result in more significant and potentially harmful impact forces. Such forces can lead to overuse injuries in gymnasts. Research led to a rule change that allowed gymnasts to adopt landing strategies that reduce impact forces without incurring score penalties. In the context of tennis, biomechanics research has explored the issue of tennis elbow (lateral epicondylitis), a common overuse injury in novice players. The research revealed that faulty technique during backhand strokes, specifically maintaining a flexed wrist during ball impact, was a potential cause of tennis elbow. Players who maintained a neutral wrist position through ball impact were found to be less susceptible to this condition. This biomechanical insight helped in preventing tennis elbow by emphasizing proper technique.
The text goes on to describe the impact of biomechanics on sports equipment design, specifically in the running shoe industry. Following a running boom in the United States in the 1970s, which led to an increase in running-related injuries, biomechanics research played a pivotal role in shaping the design of running shoes. Biomechanical tests conducted at university biomechanics laboratories provided insights into shoe performance, and some shoe companies even hired biomechanics as consultants. The need for running shoes with both cushioning and stability led to advancements in design, resulting in fewer running injuries. The section concludes by emphasizing that sport and exercise biomechanics can contribute to performance improvement while also aiding in injury prevention and rehabilitation by refining techniques, equipment, and training. The text acknowledges that most technique improvements have occurred without explicit assistance from biomechanics. However, it suggests that as more individuals within the sports community, including teachers, coaches, and athletes, become acquainted with biomechanics, the field may have a more pronounced impact on enhancing techniques.
The text also recognizes that sport and exercise biomechanics remains a relatively young field and that the number of practicing sport biomechanists is limited. Nevertheless, it emphasizes the potential of biomechanics to effect positive changes in various sports and encourages a broader application of biomechanics knowledge to enhance athletic performance, prevent injuries, and expedite rehabilitation.
The History of Sport Biomechanics: A Journey through Time
This section delves into the historical evolution of sport biomechanics, tracing its roots from the 19th century to the 21st century. It highlights how biomechanics, a field intimately intertwined with kinesiology, has progressed and become more prominent as a tool for analyzing and improving human movement in sports and exercise.
The narrative begins by distinguishing between the terms ‘kinesiology’ and ‘biomechanics.’ ‘Kinesiology,’ rooted in the late 19th century, was used to describe the study of movement, while ‘biomechanics’ only gained popularity in the 1960s. Nowadays, ‘kinesiology’ is understood as the study of human movement.
During the 20th century, an individual course in kinesiology formed an essential component of the undergraduate curriculum in physical education at numerous American institutions. However, the primary focus of these courses was often applied anatomy, with limited exposure to mechanics and physiology. This approach was sometimes insufficient to provide students, including future coaches and physical education teachers, with a practical understanding of mechanics.
The historical narrative also highlights key developments and researchers in biomechanics. Etienne Jules Marey’s work in the late 19th century, as described in ‘Le Mouvement,’ set the foundation for modern biomechanics and exercise physiology laboratories. This included the use of devices such as cameras and pressure-sensitive instruments to measure and record forces and motions in various activities, including those of humans and animals. Early indications of sport and exercise biomechanics research appeared in unexpected places, such as The Baseball Magazine in 1912, where a study measured the speed of baseball pitches.
In the 1920s and 1930s, researchers like Archibald V. Hill and Wallace Fenn delved into the mechanics and energetics of activities like sprinting. Meanwhile, Thomas Cureton, while better known for his work in exercise physiology, contributed to the understanding of swimming mechanics and techniques. This era also saw the emergence of one of the first “biomechanics” textbooks, written by Arthur Steindler in 1935.
The 1940s saw a pause in biomechanics research due to World War II, but in 1955, John Bunn’s book “Scientific Principles of Coaching” emphasized the mechanics of human movement in sports, moving away from a sole focus on anatomy.
By the 1960s, the term ‘biomechanics’ became popular, accompanied by a surge in research activities. In 1967, the First International Seminar on Biomechanics took place in Zurich, Switzerland, primarily focusing on the mechanics of human movement. This marked the start of international conferences on biomechanics, held every two years.
In 1968, the Journal of Biomechanics was first published, featuring numerous sport biomechanics papers in its inaugural volume. The 1960s also witnessed the establishment of graduate programs in biomechanics within physical education departments.
Over time, the field grew significantly, leading to the formation of several biomechanics societies, including the International Society of Biomechanics in 1973 and the American Society of Biomechanics in 1977. The early 1980s saw the creation of the International Society of Biomechanics in Sport, specifically representing sport biomechanists. Several specialized journals in the field, like the Journal of Applied Biomechanics and Sport Biomechanics, were established to cater to sport biomechanics research.
The closing section underscores the continuous growth of research and involvement in sport and exercise biomechanics, especially since the advent of modern digital computers. These technological advancements have facilitated data collection, analysis, and modeling, expanding the possibilities in biomechanics research. Consequently, courses have been adapted and expanded to include more mechanics content, and many kinesiology courses have been renamed as biomechanics courses.
The narrative concludes by addressing the initial question: Why has biomechanics not had a more profound impact on sport thus far? It attributes this to the relatively recent emergence of biomechanics and a shortage of time to accumulate a substantial impact. However, it maintains optimism, stating that as more sport and exercise professionals become familiar with biomechanics, its influence will become more pronounced. Thus, the section encourages readers to delve further into biomechanics to grasp its potential and applications.
The Organization of Mechanics: A Foundation for Biomechanics
This section serves as the gateway to the study of sport and exercise biomechanics by establishing the groundwork of mechanics. Mechanics is defined as the science that explores the effects of forces acting on objects, and this definition is expanded upon to include a broader understanding of mechanics as the science concerned with the effects of forces acting on objects.
Mechanics encompasses various branches, each designed to address distinct aspects of the physical world. These branches include rigid-body mechanics, deformable-body mechanics, fluid mechanics, relativistic mechanics, and quantum mechanics. In rigid-body mechanics, objects are treated as perfectly rigid, simplifying the analysis. In deformable-body mechanics, the deformation of objects is taken into account, making analyses more complex. Fluid mechanics is focused on the behavior of liquids and gases, while relativistic mechanics delves into Einstein’s theory of relativity, and quantum mechanics is related to quantum theory. Each branch is tailored to explain particular characteristics of the physical world.
Rigid-body mechanics, which assumes that objects are perfectly rigid and doesn’t account for bending, stretching, or compressing, is especially relevant to the study of sport and exercise biomechanics as it effectively describes the gross movements of humans and the equipment they use. Additionally, because some sports and exercises take place in fluid environments, a basic understanding of fluid mechanics is also essential.
In rigid-body mechanics, the objects under investigation are assumed to be rigid, even though, in reality, they do experience small deformations due to forces. However, these deformations generally don’t significantly affect the gross movements of the body or limbs, allowing for the consideration of the body as a system of linked rigid bodies. Nevertheless, small deformations over time can lead to overuse injuries.
Rigid-body mechanics can be further divided into statics, concerning objects at rest or in constant velocity, and dynamics, focused on objects in accelerated motion. Dynamics can be subdivided into kinematics, describing motion, and kinetics, dealing with the forces that cause or influence changes in motion.
However, to proceed effectively, readers must first acquire a solid grasp of fundamental mechanics concepts. This section serves as an introduction to the fundamental building blocks upon which sport and exercise biomechanics are based.
Basic Dimensions and Units of Measurement in Mechanics: A Foundation for Biomechanics
This section lays the foundation for understanding biomechanics, emphasizing the need for quantitative analysis and measurement in this scientific field. Biomechanics seeks to provide quantitative descriptions of human movement and its underlying causes, necessitating a standardized system of units and measurements. In this exploration of basic dimensions and units of measurement used in mechanics, the objective is to determine how to measure various parameters crucial for biomechanical analysis.
Consider observing a football player (American football) returning a kickoff. To describe this player’s runback, terms such as position on the field, distance covered, time taken, speed, and direction are essential. Additionally, factors like size, inertia, power, momentum, force, mass, weight, velocity, and acceleration come into play. These terms, though possibly unfamiliar at this stage.
To address these parameters effectively, we must identify the fundamental dimensions required for description. Length, a basic dimension, enables the measurement of space and is crucial in sports such as shot put and high jump, where how far or how high represents performance. In sports like golf or baseball, length contributes to the success determination. Athletes’ anthropometric measurements, including height and the lengths of sporting equipment, may influence performance. Sports also have specific dimensions outlined in their rules, such as field size, basket height, or race length.
Measuring length using systems like inches, feet, or yards is common in the United States, but the scientific community predominantly employs the International System of Units (SI). The SI unit for length is the meter (m), approximately equivalent to 3.28 feet or 39 inches. There are 2.54 centimeters (cm) in an inch, and 100 cm in a meter. Longer distances are often measured in kilometers (km) or miles, with 1 kilometer being equal to 1000 meters and 1 mile roughly corresponding to 1.609 kilometers.
Time is another vital dimension for describing performance in sports, particularly in races where the shortest time often defines the winner. Goalie reactions and movement times play significant roles in various team sports, and game-specific rules incorporate timing elements. The SI unit for time is the second (s).
By possessing measurements of both length and time, we gain the basic dimensions required for describing motion: space and time. Descriptors such as speed, velocity, and acceleration are derived from these dimensions. For instance, speed and velocity are expressed as units of length per unit of time, while acceleration involves units of length per unit of time squared. Some of the descriptors from the football example focus on the player’s attributes rather than motion itself, encompassing terms like momentum, power, force, inertia, mass, and weight.
Mass and Inertia: The Importance of Mass in Sport and Exercise
This section dives into the concept of inertia and its relationship with mass, emphasizing its significance in the context of sports and exercise. Inertia, a property of objects that resists changes in their motion, is the mechanical term that correlates with the everyday use of the word to indicate resistance or reluctance to change. In a mechanical context, this resistance pertains specifically to changes in motion.
To gain a deeper understanding of inertia, consider the challenge of starting or stopping the motion of different athletes: an Olympic shot-putter versus an Olympic gymnast. It’s evident that the shot-putter is more challenging to move; this athlete possesses more inertia. Therefore, it’s reasonable to assert that larger objects have greater inertia than smaller objects. However, what precisely constitutes “larger”? It might be tempting to assume that weight determines inertia since heavier objects exhibit more inertia. Yet, this assumption is not entirely accurate. Inertia is measured by mass, whereas weight signifies the force of gravity acting on an object.
Mass is the key measure of inertia, whereas weight is a measure of the gravitational force’s influence on an object. As an example, an Olympic shot-putter would weigh less on the moon than on Earth due to the moon’s lower gravitational pull. However, the athlete’s inertia—resistance to changes in motion—remains the same on both the moon and Earth.
In the context of sports and exercise, mass plays a pivotal role in situations where an athlete must initiate or arrest the motion of objects (e.g., balls or rackets), other athletes (e.g., football tackles or judo throws), or their own bodies or body parts. The mass of the object to be moved or stopped fundamentally dictates the effort required to achieve these actions.
The International System of Units (SI) employs the kilogram as its unit of measurement for mass. The kilogram is strictly a unit of measure for mass and is abbreviated as kg. In this system, a kilogram weighs approximately 2.2 pounds and is equivalent to 1000 grams.
By establishing mass as one of the three fundamental dimensions used in the SI system (alongside length and time), we lay the foundation for understanding other mechanical quantities and their units of measurement. Length, time, and mass serve as the core dimensions upon which various mechanical aspects and SI units are built. In the United States, the commonly used basic dimensions are length, time, and force, with their respective units being the foot, second, and pound. These dimensions and units form the basis of quantitative analysis and measurement in biomechanics.