Muscular System
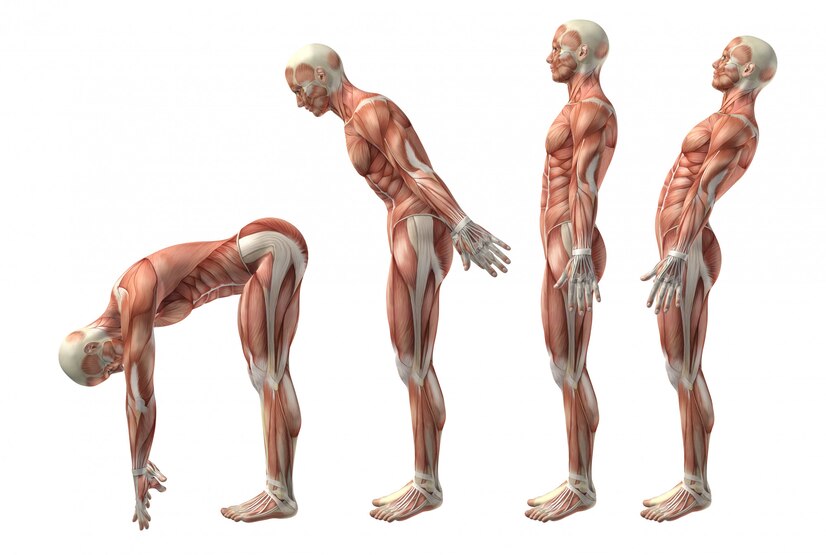
The Muscular System: Orchestrating Motion and Stability
The muscular system is a fundamental component of the human body, working in tandem with the nervous and skeletal systems to enable motion, provide stability, and execute intricate movements with precision. It serves as the intermediary between the commands of the nervous system and the structural framework of the skeletal system, allowing us to perform a vast array of physical activities, from the simplest to the most complex. This comprehensive exploration of the muscular system delves into its structure, function, and the remarkable intricacies of muscle contraction.
- The Role of Muscles in Movement Production:
- Dynamic and Versatile: Muscles are dynamic, versatile tissues that generate internal tension in response to signals from the nervous system. This internal tension is harnessed to manipulate the bones of the body, producing a wide spectrum of movements.
- Movers and Stabilizers: Muscles are not only responsible for producing movement but also for stabilizing the body. They function as active supports, ensuring proper joint alignment and balance during various activities.
- Structure of Skeletal Muscles:
- Muscle Bundles: Skeletal muscles consist of multiple bundles of muscle fibers held together by connective tissue. Each bundle is further divided into layers, from the outer surface to the innermost layer.
- Fascia and Epimysium: The muscle is enveloped by an outer layer of connective tissue called fascia and an inner layer called the epimysium. These structures are integral in forming the muscle’s tendon.
- Perimysium and Endomysium: Within each bundle, known as a fascicle, individual muscle fibers are surrounded by connective tissue called perimysium. Further, each muscle fiber within the fascicle is enveloped by endomysium.
- Importance of Connective Tissues: Connective tissues within the muscle facilitate the transmission of forces generated by the muscle to the bones, enabling motion. These layers of connective tissue extend to form the tendon, which attaches muscles to bone and acts as an anchor for exerting force.
- Muscle Fibers and Contractile Elements:
- Structural Components: Muscle fibers, the functional units of skeletal muscle, consist of various structural components, including the sarcolemma (plasma membrane), sarcoplasm (cellular plasma), nuclei, and mitochondria.
- Myofibrils and Sarcomeres: Myofibrils within muscle fibers contain myofilaments, which are the contractile components of muscle tissue. These myofilaments include actin (thin filaments) and myosin (thick filaments). Sarcomeres, the functional units of muscle contraction, are defined by their placement between two Z lines along the myofibril.
- Regulatory Proteins: Two essential proteins, tropomyosin and troponin, play critical roles in muscle contraction. Tropomyosin, located on the actin filament, blocks myosin binding sites in the relaxed state. Troponin, also situated on the actin filament, facilitates muscle contraction by providing binding sites for calcium and tropomyosin when needed.
- The Process of Muscle Contraction:
- Initiation by Nervous System: Muscle contraction is initiated by signals from the nervous system, specifically motor neurons that release neurotransmitters at the neuromuscular junction.
- Sliding Filament Theory: The sliding filament theory explains how muscle contraction occurs. During contraction, myosin filaments pull actin filaments closer together, shortening the sarcomere and, consequently, the entire muscle fiber.
- Role of Calcium: Calcium ions play a crucial role in muscle contraction by binding to troponin and enabling the movement of tropomyosin, thereby exposing myosin binding sites on actin filaments.
- ATP as an Energy Source: ATP (adenosine triphosphate) is essential for muscle contraction, as it provides the energy required for myosin heads to bind to actin and subsequently release.
Understanding the muscular system’s structure and the mechanics of muscle contraction is pivotal in grasping how our bodies execute movements and maintain stability. This knowledge serves as the foundation for exploring various aspects of muscle function, including muscle types, fiber types, and the effects of exercise on muscle health and performance.
Neural Activation: The Catalyst for Muscle Contraction
The intricate process of muscle contraction begins with neural activation, which serves as the vital communication link between the nervous system and the muscular system. Neural activation is essential for initiating and controlling muscle contractions, allowing us to perform an astonishing array of movements. In this comprehensive exploration, we delve into the neural mechanisms behind muscle activation, including the role of motor neurons, neuromuscular junctions, and the “all or nothing” law. Additionally, we unravel the sliding filament theory, a fundamental concept that elucidates the mechanics of muscle contraction.
- Motor Neurons and Neuromuscular Junctions:
- Motor Units: Motor neurons originating from the central nervous system (CNS) play a pivotal role in muscle activation. A motor unit comprises one motor neuron and the muscle fibers it innervates.
- The Neuromuscular Junction: The point of contact between a motor neuron and an individual muscle fiber is known as the neuromuscular junction. This specialized synapse, or connection, facilitates the transmission of neural signals to muscle fibers.
- Chemical Signaling: Neural activation involves the transmission of electrical impulses, also called action potentials, down the axon of the motor neuron. When these impulses reach the axon terminal, neurotransmitters, such as acetylcholine (ACh), are released.
- Neurotransmission: Neurotransmitters cross the synapse and bind to specific receptor sites on muscle fibers. ACh, the neurotransmitter involved in neuromuscular signaling, initiates a series of events that lead to muscle contraction.
- Sliding Filament Theory:
- Muscle Contraction Mechanism: The sliding filament theory elucidates how thick (myosin) and thin (actin) filaments within the sarcomere slide past one another during muscle contraction. This process ultimately shortens the sarcomere and, consequently, the entire muscle fiber.
- Force Generation: By interlocking and sliding, myosin and actin filaments generate force, resulting in muscle contraction. The sarcomere, the basic functional unit of contraction, undergoes changes in length as these filaments interact.
- Excitation-Contraction Coupling: Putting It All Together:
- Seamless Coordination: Excitation-contraction coupling is the intricate process through which neural stimulation leads to muscle contraction. It integrates neural activation and the sliding filament theory, effectively translating neural signals into mechanical force.
- Sequential Steps: This process encompasses a series of steps that begin with neural messages (neural activation) and culminate in muscle contractions.
- Motor Units and the “All or Nothing” Law:
- Motor Unit Recruitment: Skeletal muscles are divided into motor units, each comprising one motor neuron and the muscle fibers it innervates. The “all or nothing” law stipulates that either all muscle fibers within a motor unit contract maximally or none do.
- Determinants of Muscle Strength: The overall strength of a muscle contraction depends on two factors: the size of the motor units recruited (i.e., the number of muscle fibers within the unit) and the number of motor units activated simultaneously.
- Functional Correlation: The size of motor units within a muscle correlates with the specific function of that muscle. Muscles responsible for precise, fine movements have smaller motor units, whereas muscles required for powerful, gross movements have larger motor units.
Understanding the intricate neural processes that underlie muscle activation is crucial for comprehending how our bodies execute movements. This knowledge forms the foundation for exploring various aspects of muscle physiology, including muscle fiber types, neuromuscular adaptations, and the role of proprioception in motor control.
Diversity in Muscle Fiber Types:
Muscles in the human body exhibit remarkable diversity in their chemical and mechanical properties, giving rise to different muscle fiber types. These distinct fiber types, broadly classified as Type I and Type II, play crucial roles in determining an individual’s physical capabilities and performance. In this comprehensive exploration, we delve into the intricate characteristics and functions of these muscle fiber types, shedding light on their essential contributions to various aspects of human movement.
- Type I Muscle Fibers (Slow-Twitch):
- Red Fiber Characteristics: Type I muscle fibers are often referred to as “red fibers” due to their rich vascularization and myoglobin content. Myoglobin, akin to hemoglobin in red blood cells, enhances oxygen delivery to these fibers.
- Mitochondrial Abundance: Type I fibers boast a high number of capillaries and mitochondria, which are essential for the efficient conversion of food energy into ATP, the cellular fuel.
- Fatigue Resistance: Type I fibers are known for their endurance, as they exhibit resistance to fatigue. This makes them invaluable for activities requiring prolonged contractions, such as maintaining posture and stability against gravity.
- Size and Speed: These fibers tend to be smaller in diameter and are slower to produce maximal tension compared to Type II fibers.
- Type II Muscle Fibers (Fast-Twitch):
- White Fiber Characteristics: Type II muscle fibers are often termed “white fibers” due to their relatively lower vascularization, myoglobin content, and mitochondrial density compared to Type I fibers.
- Subdivision into Type IIa and Type IIx: Type II fibers are further classified into Type IIa and Type IIx based on their specific characteristics.
- Type IIx Characteristics: Type IIx fibers have a low oxidative capacity and are prone to rapid fatigue. They are designed for intense, brief bursts of power and force generation.
- Type IIa Characteristics: Type IIa fibers have a higher oxidative capacity and exhibit slower fatigue compared to Type IIx fibers. They are often referred to as intermediate fast-twitch fibers because they can utilize both aerobic and anaerobic metabolism to generate energy.
- Combinatorial Nature of Muscle Fiber Types:
- Variable Muscle Composition: It is essential to recognize that most muscles comprise a combination of slow-twitch (Type I) and fast-twitch (Type II) muscle fibers.
- Functional Adaptation: The specific blend of muscle fiber types within a muscle is tailored to its functional requirements. For instance, muscles involved in postural control may contain a higher proportion of Type I fibers to sustain prolonged contractions, while muscles dedicated to powerful movements, like sprinting, may have a larger share of Type II fibers.
Understanding the intricate mosaic of muscle fiber types in the human body provides valuable insights into physical performance, training adaptations, and athletic potential. Individual variations in muscle composition can influence an individual’s aptitude for endurance activities, strength-based tasks, and overall athletic prowess. By recognizing the unique characteristics of each muscle fiber type, individuals and fitness professionals can tailor training regimens to maximize performance and achieve specific fitness goals.
The Multifaceted Roles of Muscles in Human Movement
Muscles are the unsung heroes of the human body, orchestrating a symphony of movements that allow us to navigate our physical world. These versatile tissues serve a multitude of functions, each vital to our ability to manipulate forces, generate movement, and maintain stability. In this comprehensive exploration, we unveil the diverse roles muscles play, categorizing them as agonists, synergists, stabilizers, or antagonists, and shedding light on their indispensable contributions to human motion.
- Agonist Muscles (Prime Movers):
- Driving Force: Agonist muscles are the muscle group primarily responsible for executing a specific movement. They serve as the powerhouse behind the desired action.
- Example: In the context of hip extension, the gluteus maximus takes center stage as the agonist muscle, propelling the leg backward.
- Synergist Muscles (Supporting Cast):
- Collaborative Effort: Synergist muscles work in tandem with agonists to facilitate and enhance movement. They assist prime movers in executing a particular action.
- Example: During hip extension, the hamstring complex and the erector spinae act as synergists alongside the gluteus maximus, contributing to the seamless execution of the movement.
- Stabilizer Muscles (Foundation Builders):
- Maintaining Stability: Stabilizer muscles play a critical role in supporting and stabilizing the body while prime movers and synergists perform dynamic movement patterns.
- Example: In the lumbo-pelvic-hip complex during hip extension, the transversus abdominis, internal oblique, and multifidus muscles act as stabilizers, ensuring the integrity of the low back, pelvis, and hips.
- Antagonist Muscles (Balancing Act):
- Opposing Actions: Antagonist muscles perform actions that are opposite to those of agonist muscles. They act as a counterbalance, providing control and precision in movement.
- Example: In the context of hip extension, the psoas, a deep hip flexor, assumes the role of the antagonist, working in opposition to the gluteus maximus to modulate and refine the movement.
The Intricate Dance of Muscle Functions:
- Coordination and Harmony: Human movement is a beautifully choreographed dance involving the coordinated efforts of agonists, synergists, stabilizers, and antagonists. This orchestration ensures fluidity, control, and efficiency in our everyday activities.
- Tailoring Training Regimens: Recognizing the distinct roles of these muscle categories is paramount for fitness professionals and individuals alike. Tailoring training regimens to target specific muscle functions can lead to improved performance, injury prevention, and overall fitness gains.
- Balancing Act: Achieving a harmonious balance between these muscle functions is essential for maintaining a healthy musculoskeletal system. Imbalances can lead to dysfunction, discomfort, or injury, highlighting the importance of comprehensive training programs that address all facets of muscle function.
Understanding the intricate and multifaceted roles that muscles play in human movement is a cornerstone of effective exercise programming, physical therapy, and athletic performance optimization. By appreciating the nuanced interplay between agonists, synergists, stabilizers, and antagonists, individuals can fine-tune their training strategies and unlock their full movement potential, ultimately enhancing their quality of life.