Types of Strength Training
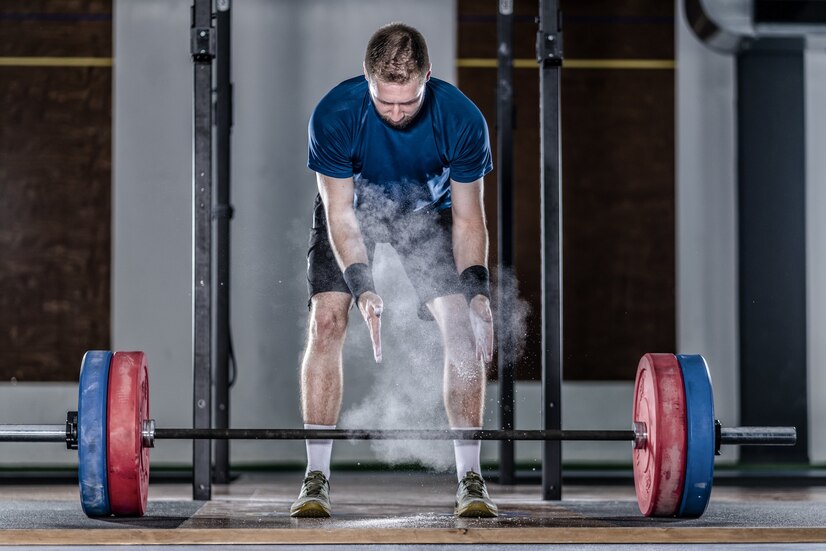
Strength Training Types and Considerations
For both athletes and fitness enthusiasts, strength training is a common component of their overall training regimens. Athletes primarily seek improvements in strength and power that can positively impact their sports performance. Fitness enthusiasts, on the other hand, may also be interested in enhancing health outcomes, including blood pressure reduction and changes in body composition that lead to a lean and fit appearance. When evaluating various types of strength training, several critical factors come into play:
- Motor Performance: Does the type of strength training lead to enhancements in motor performance? Common tests for motor performance include vertical jump tests, 40-yard sprints, and distance throwing of objects like balls or medicine balls.
- Strength Across Range of Motion and Velocities: Does the training increase strength across the full range of motion and at different movement velocities? Many athletic activities and daily movements require strength and power over a wide range of joint motion and at varying speeds.
- Body Composition Changes: To what extent does the training influence changes in body composition, such as body fat percentage or fat-free body mass?
- Expected Strength and Power Increases: How much can one anticipate increases in strength and power over a specified training period with a particular type of training?
- Comparative Analysis: How does the effectiveness of one type of training compare to others in terms of the factors mentioned above?
A substantial body of research has been conducted on various types of resistance training. However, the application of results is often limited due to the short-term nature of many studies (typically 8 to 12 weeks) and their focus on sedentary or moderately trained individuals. The ability to directly apply such findings to long-term training (spanning years) and highly trained athletes or fitness enthusiasts is questionable. Highly trained individuals may experience smaller changes in strength and body composition compared to their untrained counterparts over shorter training periods. This suggests that achieving changes in strength and body composition in athletes and advanced fitness enthusiasts is more challenging.
Other factors that affect strength gains include the training volume (the number of sets and repetitions) and training intensity (expressed as a percentage of one’s one-repetition maximum, or 1RM). The variation in these factors across different studies further complicates result interpretation. Additionally, not all muscle groups necessarily respond at the same rate or to the same extent to identical training programs, making it challenging to make broad comparisons.
Ultimately, the effectiveness of a particular type of strength training in comparison to others depends on the programs’ efficacy used in the research. For example, a comparison of an optimal dynamic constant external resistance training program against an ineffective isokinetic program would favor the former, and vice versa. While it’s ideal to have long-term comparisons using optimal programs, this isn’t always the case. However, there’s sufficient research to provide some tentative conclusions on different types of strength training and their applicability within a training program.
In the upcoming section, major research studies and their conclusions on various strength training types will be addressed. These findings can help individuals make informed decisions about their training regimens.
Isometric Training
Isometric training, also known as static resistance training, involves muscular actions where there is no visible change in the length of the total muscle and no movement at the involved joint(s). These actions can range from voluntary efforts against less than 100% of maximal voluntary muscle action (MVMA) to 100% MVMA against an immovable object.
Isometric training often involves pushing or pulling against an immovable object such as a wall or a weight machine loaded beyond an individual’s maximal concentric strength. Alternatively, it can involve having a weaker muscle group resist the movement created by a stronger muscle group, such as trying to flex the elbow with the elbow flexors while simultaneously resisting that movement by pushing down with the opposite hand. This causes the weaker muscle group to perform isometric actions at 100% MVMA. Additionally, isometric actions can be integrated into exercises after completing a partial range of motion in some cases, such as functional isometrics.
The concept of isometric training gained attention in the early 1950s when German researchers Hettinger and Muller suggested that daily 66% MVMA isometric actions performed for just six seconds could lead to weekly strength gains of 5%. These remarkable gains with minimal time and effort captured public interest.
The effectiveness of isometric training can be influenced by several factors, such as the number of muscle actions, the duration of muscle actions, their maximal or submaximal nature, and the frequency of training. Due to the simultaneous manipulation of several of these factors in most isometric training studies, it can be challenging to pinpoint the significance of each factor. Nonetheless, there’s enough research to offer recommendations and tentative conclusions about isometric training.
Maximal Voluntary Muscle Actions
Isometric strength can be enhanced through submaximal isometric muscle actions. However, there is some disagreement on the requirement for maximal voluntary muscle actions (MVMAs) to induce strength increases. Some studies suggest that MVMAs are superior to submaximal isometric muscle actions for producing strength gains, while others show no difference in strength increases between maximal and submaximal actions.
It’s worth noting that how a maximal voluntary isometric action is executed could lead to adaptational differences. The manner in which maximal force is developed, such as rapidly or over a specified time frame, may result in different adaptations. Training where maximal force is developed in a specified time frame may affect peripheral nervous system components (e.g., muscle membrane electrical activity), while training that focuses on developing maximal force as quickly as possible may impact muscle contractile properties (i.e., excitation-contraction coupling).
Isometric training quality remains an area for further investigation. Typically, MVMAs are used in training for healthy individuals, while submaximal isometric actions are common in rehabilitation or remedial strength training programs where maximal muscle actions are contraindicated.
Number of Muscle Actions and Duration
The number and duration of muscle actions play significant roles in isometric training, influencing the magnitude of strength gains. The duration of isometric actions can vary from a few seconds to longer durations, and numerous combinations can lead to notable improvements in strength.
Hettinger and Muller (1953) initially suggested that performing only one 6-second muscle action per day could lead to maximal strength gains. Subsequent research has investigated various combinations of the number and duration of both maximal voluntary muscle actions (MVMAs) and submaximal isometric actions and their impact on strength.
The majority of studies have used isometric actions lasting 3 to 10 seconds. Combining different durations and numbers of muscle actions can result in significant strength gains. For example, some studies have found strength gains from four sets of six repetitions of two-second isometric actions at 50% MVMA (adductor pollicis) or from four muscle actions, each lasting 30 seconds, at 70% MVMA (quadriceps). It’s important to note that these studies typically involved healthy but non-weight-trained participants.
The number of muscle actions and their duration individually show weaker correlations to strength gains compared to the combined effect of duration and number. In other words, total duration appears to be more crucial for strength gains. Optimal strength gains can be achieved through two main approaches: either a small number of long-duration muscle actions or a high number of short-duration muscle actions. For instance, performing seven daily one-minute muscle actions at 30% of MVMA or 42 three-second MVMAs within a single training day can both lead to approximately 30% increases in isometric MVMA.
There is also some evidence suggesting that longer-duration isometric actions may be superior in eliciting strength gains compared to shorter-duration actions. For example, in a study that trained the quadriceps at 70% of MVMA, participants who performed four 30-second isometric actions showed significantly greater increases in isometric strength compared to those who did four sets of 10 repetitions, each lasting three seconds. Interestingly, the total duration of isometric muscle actions (120 seconds per training session) was identical for both training programs. However, the longer-duration isometric actions led to significantly greater strength gains.
The reason behind this could be related to blood flow occlusion, which can occur during isometric actions and might stimulate greater strength gains. Research suggests that occlusion may lead to higher metabolite concentrations, increased acidity, and higher serum growth hormone concentrations. Training with blood flow occlusion has been found to result in increased lactate concentrations compared to training without occlusion, indicating elevated intramuscular metabolite concentrations.
Moreover, the rapidity with which force is developed during isometric actions can influence strength gains. A study that involved increasing muscle force as rapidly as possible during isometric knee extensor actions (lasting approximately one second) showed significantly greater increases in maximal voluntary muscle action (MVMA) compared to those who took four seconds to reach maximal force. This highlights the importance of force development speed in training.
In conclusion, the number and duration of muscle actions are vital factors in isometric training. Various combinations can lead to strength gains, but longer-duration isometric actions may be more effective, especially when quick strength improvements are desired. Blood flow occlusion and the speed of force development during isometric actions can also influence strength gains. Generally, a practical approach to isometric training may involve performing at least 15 MVMAs, or near MVMAs, lasting three to five seconds during three sessions per week, as discussed in the section on training frequency.
Training Frequency
Training frequency, or how often individuals engage in isometric training sessions, plays a crucial role in determining its effectiveness in increasing strength and promoting muscle hypertrophy. The frequency of isometric training sessions varies and has been a subject of investigation in scientific studies.
Three training sessions per week, utilizing either maximal or submaximal isometric actions, have consistently resulted in significant increases in isometric maximal voluntary muscle action (MVMA). These findings are supported by several studies, with training durations spanning 6 to 16 weeks. The observed increases in isometric MVMA during this period have ranged from 8% to as much as 79%. However, the question of whether three weekly training sessions cause the maximum possible strength gains is not entirely settled.
Hettinger (1961) calculated that alternate-day isometric training is approximately 80% as effective as daily training sessions, and once-a-week training is roughly 40% as effective as daily training. Moreover, training once every two weeks appears not to result in strength increases, although it can help maintain existing strength levels. It is evident that daily isometric training may be optimal for achieving maximum strength gains, but two or three training sessions per week are sufficient to bring about significant increases in maximal strength. Among these options, three sessions per week are the most frequently utilized in research studies.
Muscle Hypertrophy
Isometric training can lead to muscle hypertrophy, resulting in an increase in muscle size. In the past, muscle hypertrophy was often measured using limb circumferences. However, recent technologies such as computerized tomography, magnetic resonance imaging (MRI), and ultrasound provide more accurate measurements of muscle cross-sectional area (CSA) and thickness. These advanced techniques have been employed to investigate muscle hypertrophy caused by isometric training.
Studies have shown that isometric training can lead to significant hypertrophy in trained muscles. For example, the cross-sectional area of the quadriceps can increase by an average of 8.9% after 8 to 14 weeks of isometric training. Similarly, significant gains in the cross-sectional area of elbow flexors, up to 23%, have been reported following isometric training. These increases in CSA typically accompany improvements in maximal strength, illustrating the relationship between muscle size and strength.
The extent and occurrence of muscle hypertrophy may vary depending on the muscle group and the type of muscle fibers involved. For instance, a study reported that type I and II muscle fiber diameters in the vastus lateralis did not change after isometric training at 100% of MVMA. In contrast, the soleus exhibited approximately 30% increases in the cross-sectional area of type I and II fibers following isometric training. Similarly, only the type II fibers of the lateral gastrocnemius showed an increase in area (30-40%) after identical isometric training.
The duration of muscle actions during isometric training can also affect the degree of muscle hypertrophy. Longer-duration isometric actions have been associated with greater gains in muscle CSA compared to shorter-duration actions. For example, training the quadriceps with four 30-second actions and four sets of 10 repetitions, each lasting three seconds, resulted in a significant increase in quadriceps CSA (10-11%), while shorter-duration actions led to nonsignificant increases (4-7%) in CSA.
In addition, studies have explored the relationship between training intensity and muscle hypertrophy. It was found that maximal MVMA may induce significantly greater hypertrophy compared to 60% MVMA over a 10-week training period. The duration of isometric actions per training session was equivalent (120 seconds) between the two training programs. Notably, there doesn’t appear to be a direct relationship between training volume (expressed as the total duration of isometric actions per session or the product of training intensity times total duration) and the rate of CSA increase. This suggests that various combinations of training intensity and volume can lead to substantial muscle hypertrophy.
Furthermore, research has shown that isometric actions can stimulate muscle protein synthesis, with a significant increase observed in the soleus muscle following isometric action at 40% of MVMA to fatigue. This finding further supports the notion that isometric actions can effectively induce muscle hypertrophy in both type I and type II muscle fibers.
Joint-Angle Specificity
Isometric training has shown a concept known as “joint-angle specificity.” This means that the strength gains achieved through isometric training are most pronounced at or near the joint angle at which the training is performed. Joint-angle specificity is a well-documented phenomenon, supported by numerous studies, though some exceptions have been observed.
Several factors can influence the degree of joint-angle specificity in isometric training. These factors include the specific muscle group trained, the joint angle at which the training is conducted, and the intensity and duration of the isometric actions. It’s generally attributed to neural adaptations, such as increased recruitment of muscle fibers at the trained angle and the inhibition of antagonistic muscles at the trained angle.
The carryover of significant isometric strength increases to other joint angles can vary, ranging from 5 to 30 degrees on either side of the trained joint angle. The extent of joint-angle specificity may depend on the muscle group and the specific joint angle involved. For instance, it tends to be most pronounced when training occurs with the muscle in a shortened position (25-degree angle) and to a lesser extent when the muscle is in a lengthened position (120-degree angle). Training at the midpoint of a joint’s range of motion (80-degree angle) can result in joint-angle specificity over a broader range.
Furthermore, it’s noted that the duration and volume of isometric training can affect the degree of carryover to other joint angles. Longer-duration isometric training, achieved through more isometric actions, appears to yield greater carryover.
When it comes to dynamic power increases, isometric training at one joint angle may not consistently result in significant changes in isokinetic torque across a wide range of movement velocities. However, it has been reported that isometric training at one joint angle can lead to significant improvements in dynamic force and power, both eccentric and concentric. Isometric training can also increase peak power at various percentages of one’s maximum strength.
For achieving increased force and power across a joint’s entire range of motion, it may be necessary to perform isometric training at multiple points within that range. This means targeting specific joint angles at increments of approximately 10 to 30 degrees. It is also beneficial for the total duration of isometric training to be longer, typically involving three- to five-second actions and 15 to 20 actions per session. If isometric actions cannot be executed throughout the entire range of motion, it may be more effective to perform them with the muscle(s) in a lengthened position, rather than a shortened one. Isometric training’s joint-angle specificity can be strategically used to improve dynamic strength by targeting the “sticking point” in an exercise.
Motor Performance and Isometric Training
Maximal isometric strength and its relationship with motor performance have been a topic of interest, particularly in sports and athletic activities. Isometric strength has been found to have significant correlations with performance in various sports such as basketball, rowing, and sprinting, as well as activities like countermovement and static jumping. Additionally, it has been linked to dynamic force in exercises like the mid-thigh clean pull.
However, it’s important to note that the relationship between maximal isometric strength and dynamic performance is not always straightforward. Some studies have shown significant correlations, while others have not. A review suggested that the connection between maximal isometric strength and dynamic performance is somewhat questionable. This ambiguity is partially due to the lack of sensitivity of isometric tests to detect training adaptations resulting from dynamic activities. Furthermore, isometric tests may not consistently differentiate among athletes of varying skill levels in the same sport.
Despite these challenges, there are specific findings that shed light on the relationship between isometric strength and dynamic motor performance. For example, the rate of force development during an isometric test has demonstrated correlations with dynamic performance. Isometric force development in multi-joint movements seems to correlate with abilities such as vertical jumping and clean pulls, although these correlations are moderate in strength.
While isometric testing may not always be the ideal way to monitor changes in dynamic motor performance, it does have value in sports and activities that involve a significant amount of isometric action. In cases like rock climbing, where isometric actions are essential, isometric training and testing can be highly valuable.
It’s also worth noting that isometric training at a single joint angle may not consistently increase dynamic motor performance. The lack of improvement may be due to inconsistent changes in the rate of force or power, as well as the absence of increased limb velocity in isometric training at a single angle. Other factors, such as differences in muscle fiber recruitment patterns between isometric and dynamic actions, as well as the absence of a stretch-shortening cycle in isometric actions, may limit the impact of isometric strength gains on dynamic performance.
Isometric strength can vary across the range of motion of a movement, and the correlation between dynamic performance and isometric strength can differ significantly depending on the specific joint angle at which the isometric test is conducted. Therefore, the angle at which isometric strength should be assessed to monitor or improve dynamic motor performance remains unclear.
If isometric actions are to be used for enhancing dynamic motor performance, several suggestions can be made. First, performing isometric actions at intervals of approximately 10 to 20 degrees throughout the range of motion may facilitate the carryover of strength gains to dynamic actions. Second, since most motor performance tasks are multi-joint and multi-muscle in nature, sport-specific isometric movements, involving multiple joints and muscle groups, should be used for monitoring or improving performance. Third, if past research indicates a specific point within the range of motion that shows a high correlation between isometric strength and motor performance, isometric strength should be assessed at that point. If not, starting at the strongest point within the range of motion may be a suitable initial position for isometric strength testing. Lastly, the quick development of maximal force in isometric actions has shown potential in improving motor performance, but it should be used cautiously due to the risk of injury.
Combining Isometric Training with Other Types of Training
There’s limited information available on the effects of combining isometric training with other forms of exercise. One study combined isometric training of the elbow flexors with power training, where resistance is moved as quickly as possible at different percentages of maximal force. The results showed increased peak power, but this increase was not significantly different from what could be achieved with power training alone. A similar combination of isometric training for the knee extensors and flexors with weight training, involving a fast concentric repetition phase and a rapid eccentric phase, also resulted in increased peak power at various percentages of one-repetition maximum (1RM). Again, the increase in power was not significantly different from what could be achieved through concentric-eccentric or isometric training alone. Therefore, the limited available data suggests that combining isometric training with power-type training does not offer a clear advantage in increasing power.
Other Considerations in Isometric Training
Isometric training has been shown to have specific effects and considerations. For instance, long-term isometric training has been found to decrease resting blood pressure. However, it’s important to be aware of the potential for a Valsalva maneuver, which can occur during resistance training, leading to an exaggerated blood pressure response. This maneuver should be discouraged, as it results in higher blood pressure. The blood pressure response during isometric exercise increases with factors such as duration, intensity (percentage of maximal voluntary muscle action), and muscle mass involved. This increased blood pressure response during high-intensity, large-muscle-group isometric exercise can have implications for left ventricular function. Therefore, individuals with compromised or potentially compromised cardiovascular function, such as older trainees, should consider these factors when engaging in isometric actions.
One challenge with isometric training is that trainees may experience motivational issues, as they are not lifting or moving an actual weight. Additionally, it can be challenging to assess whether trainees are performing isometric actions at the desired intensity without feedback on force development. Visual feedback of force development, especially for unfamiliar movements, can serve as positive reinforcement and encourage greater force production during isometric actions. Electroencephalography (EMG) feedback during isometric training has shown benefits for strength gains, although its effect can vary widely. The practicality of feedback equipment in various training situations may be limited, but for optimal results with isometric training, the use of a feedback monitoring system may be warranted. This can help trainees track their progress and ensure they are training at the appropriate intensity levels.
Dynamic Constant External Resistance Training
Traditionally, the term “isotonic” was used to describe muscle actions where the muscle exerts constant tension throughout. In common resistance training exercises, like free weights and weight training machines, the force exerted by the muscles is not constant but varies with the mechanical advantage of the joints involved in the exercise and the acceleration or deceleration of the resistance. Instead, the more accurate terms to describe resistance training exercises where external resistance remains constant are “Dynamic Constant External Resistance” (DCER) and “isoinertial.” These terms signify that the weight or resistance being lifted remains constant throughout the lifting (concentric) and lowering (eccentric) phases of the exercise. They do not imply that the force developed by the muscle remains constant, which is a misconception associated with the term “isotonic.”
In resistance training machines with weight stacks or plates, the external resistance has a constant value. However, the point at which a cable or strap attaches to a movable handle or foot pad on the machine changes the muscular force required to move the resistance throughout the exercise’s range of motion. Even though the muscular force required changes, the machine is still categorized as DCER or isoinertial if it has circular pulleys. With free weights and weight training machines, the external resistance or weight lifted remains constant, even though the muscular force varies during the exercise. Hence, “DCER” and “isoinertial” more accurately describe this type of resistance training, as opposed to the older term “isotonic.”
Number of Sets and Repetitions in DCER Training
The optimal number of sets and repetitions required for DCER exercises to produce maximal improvements in strength, power, body composition, and other aspects has been a subject of interest among personal trainers, strength coaches, and sports scientists. The search for this optimal number assumes certain factors, including that a universal optimal number of sets and repetitions exists, which is effective for all individuals, exercises, muscle groups, and training levels. Additionally, it’s assumed that this optimal number will lead to maximal improvements in strength, power, endurance, and body composition for an indefinite period. This would imply that training periodization and different programs for various age groups or training levels are unnecessary. However, it’s important to consider factors like pretraining status and the duration of training, which significantly impact the results of weight training programs. These factors make interpreting studies and drawing conclusions about long-term training effects challenging.
Many studies on DCER have involved novice, college-aged participants and relatively short training durations (typically 8 to 12 weeks, with some studies lasting 20 to 36 weeks). Pretraining status and training duration significantly affect the outcomes of any weight training program. Common to the majority of studies on DCER is the use of sets taken to volitional fatigue or the use of resistance based on one’s repetition maximum (RM) at some point during the training program. The idea that various combinations of sets and repetitions can increase strength is supported by research. Different combinations of non-periodized sets and repetitions, ranging from 1 to 6 sets and 1 to 20 repetitions per set, have all resulted in increased strength. Thus, there is no single optimal combination of non-periodized sets and repetitions for increasing strength.
Direct comparisons have confirmed that there is no one-size-fits-all optimal combination. For example, no significant difference in 1RM increases were found between training programs consisting of five sets of three repetitions at 3RM, four sets of five at 5RM, or three sets of seven at 7RM. Likewise, there was no significant difference between programs using various combinations of non-periodized sets and repetitions.
Training Frequency in Resistance Training
The term “training frequency” refers to the number of training sessions per week where a specific muscle group is trained or a particular exercise is performed. It plays a vital role in determining the overall training volume. For instance, the way training frequency is defined becomes crucial when comparing different training programs. Two examples include an upper-and-lower body split program versus a total-body weight training routine. Both programs may involve the same exercises, sets, and repetitions, but differ in the way training sessions are distributed throughout the week. Understanding training frequency’s definition is essential to avoid misconceptions and accurately evaluate training programs.
The optimal training frequency can depend on various factors, such as the muscle group being trained and the individual’s training status. For major muscle groups, the American College of Sports Medicine recommends a training frequency of two or three sessions per week. However, research has shown variations in optimal training frequency for different muscle groups. For instance, arm and leg musculature tend to benefit from a frequency of three sessions per week, while lumbar extension or torso rotation strength can be achieved effectively with one or two sessions per week.
There is no one-size-fits-all answer when it comes to the optimal training frequency, and it may vary based on factors like training volume and an individual’s training status. The choice of frequency can influence the overall gains in strength, power, body composition, and fitness. The majority of research studies have primarily involved novice subjects and shorter training durations, making it challenging to draw definitive conclusions. However, based on available information, novice trainees can benefit from a total-body program two or three days per week. Intermediate trainees can consider a total-body program three days per week or a split-body routine four days per week. Advanced lifters may require training four to six days per week, using various split routines to target different muscle groups in each session.
Meta-analyses of studies focusing on dynamic constant external resistance training have indicated that the optimal training frequency varies with training status and training volume. For untrained individuals, a training frequency of three days per week per muscle group appears to be optimal. Recreational nonathletes and trained athletes may find two days per week per muscle group to be more suitable. This difference is partly attributed to the higher training volumes used in studies involving trained subjects.
While these recommendations provide valuable guidelines, it’s important to note that individual responses to training can vary. Therefore, personal preferences, goals, and recovery abilities should also be taken into consideration when determining the ideal training frequency.
Motor Performance Enhancement through Dynamic Constant External Resistance (DCER) Training
Dynamic Constant External Resistance (DCER) training has been known to enhance motor performance across various tests and exercises. Several studies have reported significant, albeit relatively small, increases in a range of motor performance assessments as a result of DCER training:
- Vertical jump ability
- Standing long jump
- Shuttle run
- T-agility test
- Short sprint
- Baseball throwing velocity
- Soccer kick and ball velocity
- Shot put
However, some studies have shown statistically insignificant changes in tests such as short sprint time and vertical jump ability, underlining the potential variability in training outcomes. The degree of change in motor performance tests can be influenced by factors such as the initial physical condition of the trainee, training history, the type of weight training program, and the duration of training.
The effectiveness of DCER training on motor performance can also vary depending on the type of program used. For instance, untrained women demonstrated more significant improvements in vertical jump power and sprint ability when following a multiple-set periodized program compared to a single-set-to-momentary-fatigue program. Similarly, collegiate tennis athletes experienced better vertical jump height and tennis serve velocity with a multiple-set periodized program than with a single-set-to-momentary-fatigue program. This highlights that the structure of the training program plays a crucial role in determining the magnitude of motor performance improvements.
Other program variables, such as rest periods between sets, can also impact motor performance outcomes. Shorter rest periods may enhance repeat cycle sprint ability but may not lead to the same degree of strength increases as longer rest periods.
It’s important to note that training smaller muscle groups can still positively influence motor performance. For example, focusing on the toe and finger flexors in a training program led to significant improvements in vertical jump and shot put ability in college-age subjects.
Additionally, achieving meaningful gains in motor performance requires training all the relevant muscle groups involved in a specific task. The weakest muscles involved may limit the practical application of increased strength and power. Moreover, proper technique for the specific motor task must be trained alongside strength and power development, as technique can also influence the effective application of enhanced physical capabilities.
The implication is that, while DCER training can significantly improve motor performance ability, the specific characteristics of the training program, including exercise selection, sets, repetitions, and rest intervals, can significantly affect the degree of improvement. Tailoring training programs to match individual goals and specific motor performance tasks is essential for optimizing results.
Strength Changes in Dynamic Constant External Resistance (DCER) Training
Strength increases are a well-documented result of DCER training in both men and women. DCER exercises, such as the bench press and leg press, have shown significant improvements in 1RM (one-repetition maximum) ability for various muscle groups.
Women typically experience substantial increases in 1RM bench press and leg press ability after DCER training, with the degree of improvement varying based on factors like pretraining fitness, training duration, and program type. Strength gains range from 8% to 47% for the bench press and 8% to 148% for the leg press.
Similarly, men benefit from DCER training, with strength increases ranging from 3% to 44% in the bench press and 7% to 71% in the leg press. Again, the extent of improvement depends on individual factors, including training status and program design.
Body Composition Changes in DCER Training
Short-term DCER exercise can lead to modest changes in body composition. These changes typically involve small increases in fat-free mass and minor reductions in percent body fat, often resulting in little to no change in total body weight. These alterations are a result of concurrent increases in fat-free mass rather than substantial reductions in fat mass.
Safety Considerations in DCER Training
Safety precautions must be taken when performing DCER exercises with free weights, necessitating the use of appropriate spotting techniques. For exercises utilizing machine-based DCER, spotting is generally not required. Learning proper lifting techniques is crucial, especially for multijoint or multi-muscle-group exercises that require control in multiple planes of movement. More time may be needed to acquire these skills, compared to using machines.
Variable Resistance Training
Variable resistance equipment offers resistance that changes throughout an exercise’s range of motion. This equipment aims to match the strength curve of an exercise, which represents how strength varies across different joint angles.
Three primary types of strength curves exist: ascending, descending, and bell-shaped. While the curves shown in illustrations may appear linear, they are usually curvilinear in practice. Exercises with ascending curves, like squats and bench presses, allow for lifting more weight in the latter portion of the concentric phase. In contrast, exercises with descending curves, like upright rows, may allow for lifting more weight in the first portion of the concentric phase. Bell-shaped strength curves, found in single-joint exercises like arm curls, enable lifting more resistance in the middle portion of the range of motion.
Variable resistance machines aim to match these strength curves to optimize muscle force production. However, they may struggle to accommodate the full range of strength curve variations among individuals due to differences in limb length, tendon attachment points, and body size. Biomechanical research has demonstrated that most variable resistance equipment struggles to effectively match the strength curves of specific exercises. These issues may result in ineffective resistance at extreme ranges of motion and less curvilinear matching compared to actual isometric strength curves.
The effectiveness of variable resistance equipment in matching strength curves may vary across exercises, populations, and manufacturers. Research suggests that many variable resistance machines, especially those with cam systems, may not consistently match the strength curves of various exercises or individuals. While some equipment may perform better with certain populations, the variability in matching the strength curve remains a limitation of this training method.
Number of Sets and Repetitions in Variable Resistance Training
Variable resistance training, with durations ranging from 4 to 18 weeks, has demonstrated substantial increases in strength across a wide range of muscle groups. Various combinations of sets and repetitions have been effective in yielding significant strength gains. These protocols are typically denoted as (sets x repetitions x RM):
- 1 x 3 x 6-10RM
- 1 x 3 x 7-10RM
- 1 x 3 x 8-12RM
- 1 x 3 x 10-12RM
- 1 x 3 x 12-15RM
- 2 x 3 x 10-12RM
- 2 x 3 x 12 at 50% of 1RM
- 2 or 3 x 3 x 8-10RM
- 3 x 3 x 6RM
- 3 x 3 x 8-12RM
- 3 x 3 x 15RM
- 6 x 3 x 15-20RM
- 3 x 3 x 10RM for three weeks, 3 x 3 x 8RM for three weeks, and 3 x 3 x 6RM for six weeks
- Four sets with increasing resistance and repetitions decreasing from eight to three in a half-pyramid program
Moreover, variable resistance training has also been found to enhance maximal isometric strength throughout an exercise’s full range of motion. Therefore, multiple combinations of sets and repetitions can lead to significant strength improvements.
Strength Changes in Variable Resistance Training
Variable resistance training has shown to be effective in producing substantial increases in strength. For instance, after 16 weeks of training, males experienced a 50% increase in upper-body strength and a 33% increase in lower-body strength, while females demonstrated a 29% increase in upper-body strength and a 38% increase in lower-body strength. Increases in bench press and leg press strength have been noted in tests utilizing variable resistance equipment. This form of resistance training has been shown to lead to significant strength gains in various types of muscle actions.
Variable Variable Resistance in Training
One type of variable resistance equipment allows adjustments to the resistance curve of an exercise. Variable variable resistance equipment enables exercises to be performed with ascending, descending, and bell-shaped strength curves. This equipment concept aims to challenge muscles by utilizing different strength curves, which do not necessarily align with the exercise’s natural strength curve. Additionally, variable variable resistance equipment provides the flexibility to reduce force requirements in specific parts of an exercise’s range of motion, particularly after certain types of injuries.
A study involving women performing a total-body training program for 14 weeks, consisting of three sessions per week, illustrated significant improvements in 1RM strength, increased lean soft tissue, and decreased body fat percentage. Participants performed one set of 10 repetitions for each of the strength curves, resulting in three sets of each exercise, with the strength curves being bell-shaped, ascending, and descending. This type of equipment has proven effective in enhancing strength and promoting changes in body composition.
Motor Performance in Variable Resistance Training
There is limited information available regarding changes in motor performance resulting from variable resistance training. An example involves American football players participating in a combined program consisting of in-season football training and total-body variable resistance strength training. This program showed modest improvements in the 40-yard sprint and vertical jump ability when compared to a control group performing only the in-season football training program. However, the study did not address whether these changes were statistically significant or if there was a significant difference between the groups. While it indicated a slight increase in motor performance with variable resistance training, it doesn’t offer definitive evidence regarding the effectiveness of variable resistance training in terms of motor performance improvements compared to other training methods.
An interesting comparison was made between a cam-type variable resistance machine and an increasing lever arm–type variable resistance machine. The study involved participants performing knee extensions and leg presses, each for one set of 12 repetitions to failure. The results showed that both groups experienced increases in static leg strength. The mean vertical jump of the cam-type group increased by 0.3 inches (0.76 cm), while the lever arm–type group exhibited a significantly greater increase of 1.1 inches (2.8 cm). This finding indicates that motor performance can improve as a result of variable resistance training, and the extent of improvement depends, in part, on the specific training protocol and equipment used.
Body Composition Changes in Variable Resistance Training
Variable resistance training has been associated with significant increases in muscle thickness in the quadriceps and hamstring muscles. Moreover, increases in fat-free mass and reductions in percent body fat have been reported as outcomes of variable variable resistance training. These changes in body composition are comparable in magnitude to those observed in dynamic constant external resistance (DCER) training.
Safety Considerations in Variable Resistance Training
In terms of safety, variable resistance or variable variable resistance machines do not pose major concerns, and a spotter is typically not required. However, as with any weight training machine, it is crucial to ensure that the equipment fits the trainee correctly and that the trainee is properly positioned on the machine. Proper fit and positioning are essential for maintaining proper exercise technique and reducing the risk of injury.
Isokinetic Training
Isokinetic training refers to a specific type of muscular action performed at a constant angular limb velocity. Unlike other forms of resistance training, isokinetic training doesn’t specify resistance levels. Instead, it focuses on controlling the velocity of movement. During an isokinetic movement, acceleration occurs from 0 degrees per second until the set velocity is reached. Once that velocity is achieved, further acceleration is impossible, and any force applied against the equipment leads to an equal reaction force. The reaction force matches the force applied to the equipment throughout the exercise’s range of motion, except at the beginning and end, where acceleration and deceleration take place. This means that theoretically, muscles can exert a continual maximal force throughout most of the movement’s range, except during these acceleration and deceleration phases.
Most isokinetic equipment available in resistance training facilities allows for concentric-only actions, although some can accommodate eccentric and coupled concentric–eccentric isokinetic actions. For this discussion, we will focus on concentric-only isokinetic training. The advantages of isokinetic training include the ability to exert maximal force over a significant portion of the exercise’s range, train across various movement velocities, and minimal soreness in muscles and joints. Many isokinetic machines primarily allow for single-joint movements (e.g., knee extension or elbow flexion) and are often designed for unilateral actions (one leg or arm at a time) rather than bilateral actions (both arms or legs). However, a significant criticism of this type of training is that isokinetic muscle actions do not replicate real-world situations, which limits the applicability of isokinetic training to daily life and sport activities.
Strength Changes in Isokinetic Training
Research in concentric-only isokinetic training is predominantly of short duration, typically spanning 3 to 16 weeks. These studies usually examine strength changes in single-joint movements and test for gains using a variety of methods, including isometric, dynamic constant external resistance (DCER), eccentric-only isokinetic, and concentric-only isokinetic tests. The data gathered from these studies show that various programs, encompassing sets of different lengths, movement velocities, and repetitions, produce significant increases in strength.
There are alternative approaches to isokinetic training that result in strength gains. For example, performing as many repetitions as possible within a fixed period has proven effective. Studies have shown significant strength gains with protocols such as:
- One set of 6 seconds at 180 degrees per second
- One set of 30 seconds at 180 degrees per second
- Two sets of 20 seconds at 180 degrees per second
- Two sets of 30 seconds at 60 degrees per second
- Two sets of 30 seconds at 120 or 300 degrees per second
- One set of 60 seconds at 36 or 180 degrees per second
Additionally, strength gains can be achieved by performing maximal voluntary muscle actions until a specific percentage of peak force can no longer be generated, such as 60%, 75%, or 90% of peak force at different velocities, including 30, 60, and 90 degrees per second.
Studies on isokinetic velocity spectrum training have shown that performing several sets consecutively at various movement velocities can lead to significant power and torque gains. The order in which fast and slow velocity sets are performed can influence the type of gains observed, with some protocols showing greater power gains when faster velocities are performed first.
Eccentric-only, concentric-only, and coupled eccentric–concentric isokinetic training can result in substantial strength gains. Most of these studies indicate contraction specificity, meaning that concentric training results in greater concentric strength gains, and eccentric training yields greater eccentric strength gains. However, there are exceptions, as not all studies consistently show a high degree of contraction specificity.
In conclusion, isokinetic training can lead to significant strength gains, especially when tailored to specific protocols, even though it may not exactly replicate real-world muscle actions.
Number of Sets and Repetitions in Isokinetic Training
When it comes to determining the optimal number of sets and repetitions for concentric-only isokinetic training, research has been relatively limited. Few studies have delved deeply into this area. For instance, one study compared 10 sets of 6-second duration with as many repetitions as possible (approximately three reps) to two sets of 30-second duration with as many repetitions as possible (approximately ten reps) when training at 180 degrees per second. Surprisingly, there was no significant difference in peak torque gains between these two training protocols (Lesmes et al. 1978).
Another study examined various combinations of repetitions (5, 10, and 15) at slow, intermediate, and fast velocities of movement. The researchers found no significant strength differences after nine weeks of training three days a week (Davies 1977). Similarly, a comparison between 5 sets of 5 repetitions and 15 sets of 10 repetitions at 60 degrees per second showed minimal differences, with both groups showing significant strength improvements at various test velocities (Cirello, Holden, and Evans 1983).
These studies collectively suggest that various numbers of repetitions per set and sets can lead to significant increases in peak torque over short training durations. Additionally, it appears that, similar to dynamic constant external resistance (DCER) training, multiple sets result in significantly greater strength gains than a single set. In one study, three sets at 60 degrees per second resulted in significantly greater strength increases compared to one set at the same velocity (17% vs. 2%) when peak torque was tested at the training velocity (Kelly et al. 2007).
Training Velocity in Isokinetic Training
The optimal training velocity for concentric-only isokinetic training is a topic that has been explored in research. This depends largely on the concept of velocity specificity, which suggests that strength gains from training are greatest at the training velocity itself. The majority of research supports velocity specificity, even after very short training periods (Coburn et al. 2006). In practical terms, this means that if strength at a specific velocity of movement is needed, training should be conducted at that velocity.
Several studies have investigated the question of whether training at a faster or slower velocity is optimal. One early study indicated that two training velocities displayed some degree of velocity specificity, with faster training showing less specificity but more consistent strength gains across a range of velocities. Both training velocities in this study were relatively slow (Moffroid and Whipple 1970).
Other research suggests that the optimal training velocity may be different depending on the goal. For example, one study showed an advantage for intermediate velocity (179 degrees per second) in improving average power across various movement velocities (Kanehisa and Miyashita 1983b). Another study by the same researchers indicated velocity-specific power gains after training at either 73 or 157 degrees per second (Kanehisa and Miyashita 1983a).
In a study comparing training at 60 and 240 degrees per second, both groups improved significantly at various test velocities, but the 240-degree-per-second training group showed superior overall strength gains (Jenkins, Thackaberry, and Killian 1984).
A comparison of different training velocities and sets and repetitions indicated that each group showed its greatest gains at its specific training velocity. This suggests that the velocity of training is partly determined by the desired velocity for peak torque increases. However, there is substantial carryover to other velocities, especially those slower than the training velocity. This implies that training at a given velocity can improve strength at other velocities as well (Coyle et al. 1981).
Some research suggests that there may not be a strong reason to favor a particular velocity when seeking gains in strength. For example, training at 60 or 180 degrees per second resulted in equal gains in peak torque at various velocities (Bell et al. 1989; Lacerte et al. 1992). Training at 60 or 240 degrees per second resulted in equal isometric strength gains (Mannion, Jakeman, and Willan 1992).
Research on the optimal training velocity for eccentric isokinetic training is more limited but suggests that fast eccentric training can result in greater strength gains compared to slow eccentric training. For example, studies have shown that training at 210 degrees per second leads to greater strength gains compared to training at 20 degrees per second (Shepstone et al. 2005). Similarly, training at 180 degrees per second results in greater overall strength gains than training at 30 degrees per second (Farthing and Chilibeck 2003). These findings indicate that when focusing on eccentric training, faster training velocities may be more advantageous.
Velocity Specificity and Strength Carryover in Isokinetic Training
The concept of velocity specificity in isokinetic training, which suggests that strength gains are greatest at the training velocity, raises the question of how much these strength increases carry over to velocities other than the training velocity. Several studies have explored this aspect.
One study comparing concentric training at 36 and 108 degrees per second found that significant increases in peak torque carry over only at movement speeds slower than the training velocity (Moffroid and Whipple 1970). Similarly, a group training at 90 degrees per second showed significant increases in peak torque at 90 and 30 degrees per second but no significant increase at 270 degrees per second (Seger, Arvidsson, and Thorstensson 1998).
Another study examining concentric strength gains at velocities from 60 to 240 degrees per second suggested that there is carryover of peak torque gains at velocities both above and below the training velocity (Ewing et al. 1990). These studies collectively indicate that significant gains in concentric peak torque can occur above and below the training velocity, except when the training velocity is very slow (30 degrees per second). The greatest strength gains still tend to happen at the training velocity.
When interpreting these findings, it’s important to note that these studies considered peak torque irrespective of the joint angle at which peak torque occurred. Muscle tension control at a specific angle or muscle length could be a critical factor in how these changes affect real-world movements.
An interesting observation is that while peak torque at various velocities is generally higher than joint-angle-specific torque at a particular joint angle, there may be variation based on muscle fiber composition. Groups with different percentages of type II muscle fibers show differences in torque-velocity curves for angle-specific torque, indicating that muscle fiber type might have a greater impact on angle-specific torque than peak torque (Yates and Kamon 1983).
Additionally, research on concentric velocity specificity and carryover, when considering both peak torque and angle-specific torque, doesn’t necessarily conflict. Studies have demonstrated that fast-velocity training, especially in the range of 108 to 240 degrees per second, results in significant increases in torque below and, in some cases, above the training velocity. The magnitude of carryover to other velocities may be influenced by how fast velocities were defined in each study.
Studies indicate that slow-velocity training (36 to 96 degrees per second) leads to significant carryover in torque below and above the training velocity. However, regardless of fast- or slow-velocity training, carryover to substantially faster velocities than the training velocity appears to be less evident.
An examination comparing training velocities of 60 degrees per second (slow), 179 degrees per second (intermediate), and 300 degrees per second (fast) showed that an intermediate training velocity resulted in greater carryover of average power to a broader range of velocities above and below the training velocity compared to slow or fast training velocities (Kanehisa and Miyashita 1983b). This indirectly supports the idea that an intermediate concentric training velocity offers better carryover to velocities other than the training velocity.
In the realm of eccentric isokinetic training, research is more limited. Two studies suggest that training with fast eccentric velocities (180 and 210 degrees per second) results in greater strength gains and carryover to velocities lower than the training velocity compared to slow eccentric velocities (20 and 30 degrees per second) (Farthing and Chilibeck 2003; Shepstone et al. 2005). These studies, however, did not test peak torque above the fast training velocity, indicating that, similar to concentric training, strength gains due to eccentric isokinetic training carry over to velocities lower than the training velocity.
Body Composition Changes in Isokinetic Training
Concentric-only isokinetic training has been studied in the context of body composition changes. It has been reported to significantly increase muscle fiber cross-sectional area (Coyle et al. 1981; Ewing et al. 1990; Wernbom, Augustsson, and Thomee 2007) and total muscle cross-sectional area (Bell et al. 1992; Housh et al. 1992; Narici et al. 1989). However, some studies have shown nonsignificant changes in muscle fiber area (Akima et al. 1999; Colliander and Tesch 1990a; Costill et al. 1979; Cote et al. 1988; Seger, Arvidsson, and Thorstensson 1998) and total muscle cross-sectional area (Akima et al. 1999; Seger, Arvidsson, and Thorstensson 1998). Interestingly, some research indicates that these changes can occur in one muscle group, such as the quadriceps, and not in another, like the hamstrings, after the same concentric-only isokinetic training program (Petersen et al. 1990).
Additionally, concentric-only isokinetic training has been associated with an increase in fascicle angle, suggesting muscle hypertrophy (Blazevich et al. 2007).
Eccentric-only isokinetic training has also been studied regarding body composition changes. Similar to concentric training, eccentric training leads to increases in muscle fiber cross-sectional area and total muscle cross-sectional area (Seger, Arvidsson, and Thorstensson 1998; Wernbom, Augustsson, and Thomee 2007). Notably, fast eccentric isokinetic training at 180 and 210 degrees per second has been found to result in greater muscle and muscle fiber cross-sectional area increases compared to slow eccentric isokinetic training at 20 and 30 degrees per second and fast and slow concentric isokinetic training at 180 and 30 degrees per second (Farthing and Chilibeck 2003; Shepstone et al. 2005). This indicates that both concentric-only and eccentric-only isokinetic training can increase muscle fiber and muscle cross-sectional areas, contributing to increased fat-free mass. However, the extent of these changes may vary between different training programs.
These findings suggest that isokinetic training can lead to significant changes in body composition, such as increased fat-free mass and decreased percent fat. These changes are of a similar magnitude to those induced by other forms of training, making isokinetic training a valuable option for those seeking to alter their body composition.
Motor Performance in Isokinetic Training
Isokinetic training has shown positive effects on motor performance, encompassing a range of abilities such as vertical jump, standing broad jump, sprinting, soccer ball kicking distance, tennis serve ball velocity, and cycling power output.
Studies have demonstrated that concentric-only isokinetic training can improve various aspects of motor performance. For example, vertical jump ability (Augustsson et al. 1998; Blattner and Noble 1979; Oteghen 1975; Smith and Melton 1981), standing broad jump ability (Smith and Melton 1981), 40-yard sprint ability (Smith and Melton 1981), soccer ball kicking distance (Young and Rath 2011), and ball velocity of a tennis serve (Ellenbecker, Davies, and Rowinski 1988) have all been shown to improve with this type of training. Power output during 6-second and 30-second maximal sprint cycling also improves with concentric isokinetic training (Bell et al. 1989; Mannion, Jakeman, and Willan 1992).
Even functional abilities related to daily life, like stair climbing, speed walking, and rising from a chair, have shown improvement in individuals aged 41 to 75 years after both concentric-only and coupled concentric–eccentric isokinetic training, with the latter showing more pronounced effects (Gur et al. 2002). However, it’s worth noting that isokinetic training is usually limited to single-joint exercises, which may not universally enhance motor performance in all tasks.
The speed of isokinetic training appears to influence its effects on motor performance. Fast-speed concentric isokinetic training has been found to be more effective than slow-speed training in increasing certain aspects of motor performance (Smith and Melton 1981). For instance, one study compared fast-speed and slow-speed training for vertical jump, standing long jump, and sprinting, and the fast-speed group showed greater improvements in these areas. However, when it comes to increasing sprint cycling power output, the speed of isokinetic training may not make a significant difference (Bell et al. 1989; Mannion, Jakeman, and Willan 1992).
Additional Considerations in Isokinetic Training
Isokinetic training is known for causing minimal muscle soreness following sessions (Atha 1981). Additionally, compared to coupled concentric–eccentric isokinetic training, concentric-only training has been found to result in greater decreases in subjective pain evaluation during everyday tasks (Gur et al. 2002). This could be particularly advantageous for individuals seeking to reduce discomfort during daily activities.
Notably, concentric isokinetic training can lead to significant strength gains, particularly in the quadriceps, even after a short training duration (Coburn et al. 2006; Cramer et al. 2007). Rapid strength gains have been reported, especially in rehabilitation settings.
Another benefit of isokinetic training is its reduced risk of injury. Since there are no free weights or weight stacks to lift, the likelihood of injury is minimal, and a spotter is not required. However, to ensure optimal effort and motivation during training, it’s essential to have an accurate feedback system, such as visible force generated or work performed, which can be displayed to the exerciser. Some individuals may struggle with motivation during isokinetic training since it lacks the visual movement of traditional weights or stacks.
Eccentric Training: A Comprehensive Overview
Eccentric training, often referred to as negative resistance training, is a specialized and valuable approach in resistance training. It places a particular emphasis on the eccentric phase of muscle contractions, where the muscle lengthens as opposed to shortens. The eccentric phase plays a crucial role in various everyday activities, such as walking down stairs, which necessitates the use of the thigh muscles in eccentric actions to control the descent.
During normal dynamic constant external resistance (DCER) training, when lifting weights, muscles primarily undergo concentric contractions, shortening as they work against the resistance. However, during the lowering phase of a repetition, the same muscles are actively lengthened in a controlled manner, undergoing eccentric contractions. This eccentric phase is critical because it prevents the weight from simply falling due to gravity.
Eccentric training involves deliberately focusing on the eccentric phase of the movement and can be conducted in several ways:
- Accentuated Eccentric Training: This technique involves using resistance greater than what an individual can lift concentrically, potentially exceeding their one-repetition maximum (1RM). This is sometimes referred to as accentuated eccentric training or negative accentuated training. The accentuated eccentric phase allows for a unique and challenging stimulus for muscle growth and strength development.
- Resistance Machines: Many resistance training machines are designed to facilitate eccentric training. In these exercises, you can lift a resistance greater than the 1RM of one arm or leg and then lower it with only one limb. Some machines even allow the eccentric phase to be performed with resistance greater than the concentric phase, though not necessarily exceeding the 1RM.
- Free Weights: Eccentric training can also be achieved with free weights. In this case, spotters may add weight after the weight is lifted or apply resistance during the eccentric phase of a repetition. Alternatively, spotters can assist with lifting a resistance heavier than the 1RM, allowing the lifter to focus on the eccentric portion of the repetition. Weight release hooks can be employed to safely handle heavier resistances than the 1RM.
Safety is of paramount importance in eccentric training, especially when using free weights or non-isokinetic machines. The temptation to use more weight than can be safely controlled during the eccentric phase must be avoided. For free weight exercises like bench presses and squats, safety can be enhanced by setting the pins of a power rack to catch the weight if necessary, preventing accidents and injuries.
Eccentric training is a versatile and effective method for improving muscular strength and control. It can be tailored to various fitness levels, from beginners to advanced athletes, and its focus on the eccentric phase of muscle contractions adds a unique dimension to resistance training. Incorporating eccentric training into a comprehensive fitness routine can lead to impressive results in muscle development and strength enhancement.
Strength Changes in Eccentric Training: A Detailed Analysis
Eccentric training, often referred to as negative resistance training, is a unique and effective approach to resistance training. It primarily focuses on the eccentric phase of muscle contractions, where muscles lengthen while working against resistance. Understanding how strength changes occur during eccentric training is crucial to appreciate its significance in the fitness and training world.
- Eccentric vs. Concentric Training: In traditional dynamic constant external resistance (DCER) training, which combines both concentric (shortening) and eccentric (lengthening) muscle actions, it has been observed that greater strength increases are achieved when compared to concentric-only resistance training for the same number of repetitions (Dudley et al. 1991). This highlights the importance of incorporating an eccentric component into training routines, especially for muscle groups like the legs.
- Eccentric-Only Training: Eccentric-only DCER training, which involves exclusively working on the eccentric phase of muscle contractions, has shown remarkable results in increasing maximal strength. For instance, training with three to five sets of six repetitions at 80% of the eccentric 1RM resulted in a significant 29% increase in maximal eccentric 1RM (Housh et al. 1998).
- Total-Body Eccentric Training: An eccentric-only DCER program that includes six different exercises has demonstrated significant and similar 1RM increases, ranging from 20% to 40%, when performed by previously untrained women with either 125% or 75% of the concentric 1RM. In this case, no significant difference was observed between the two groups for strength gains (Schroeder, Hawkins, and Jaque 2004).
- Eccentric Training in Seniors: Eccentric-only DCER training in seniors, even those aged 74 years old, has been found to be effective. Training at 80% of the DCER 5RM resulted in improvements in isokinetic eccentric and isometric force capabilities, although there were no significant increases in isokinetic concentric force capabilities (Reeves et al. 2009).
- Variation in Eccentric Training: Some studies have explored variations in eccentric-only training. For example, training in an eccentric-only manner with three sets of 120 to 180% of maximal isometric strength, following a linear periodization style for three weeks, significantly increased maximal isometric strength (Colduck and Abernathy 1997).
- Comparison of Training Modes: When comparing DCER concentric-only and eccentric-only training, little difference is observed between these training modes. For example, studies have shown that both concentric-only and eccentric-only training can lead to significant improvements in isometric and concentric strength (Johnson et al. 1976). Similarly, a 20-week study with four sets of 10 repetitions demonstrated little advantage in either training mode. However, it is noteworthy that there was no significant difference in isometric strength, and the eccentric-only mode showed significant strength gains in a greater number of velocities when assessing concentric isokinetic strength (Smith and Rutherford 1995).
- Eccentric Isokinetic Training: When it comes to isokinetic training, research has yielded mixed results. Some studies have shown that eccentric-only training significantly increases isokinetic eccentric strength, while others did not find significant differences between concentric and eccentric training modes, especially at different velocities (Hortobagyi et al. 1996, Hawkins et al. 1999, Seger, Arvidsson, and Thorstensson 1998).
Accentuated Eccentric Training: A Comprehensive Overview
Accentuated eccentric training, a specialized resistance training technique, involves using more resistance during the eccentric phase of repetitions than in the concentric phase. This training method has garnered interest and scrutiny to determine its effects on strength gains when compared to normal Dynamic Constant External Resistance (DCER) training.
- Training Protocol: Accentuated eccentric training can be performed on certain machines and with specialized devices that allow for the release of weight from a barbell at the initiation of the concentric repetition phase. The key question here is whether accentuated eccentric training results in greater strength gains than conventional DCER training.
- Acute Strength Effects: In a study involving moderately trained males (Doan et al. 2002), performing accentuated eccentric DCER repetitions with 105% of the normal 1RM immediately before 1RM bench press attempts resulted in a significant increase in 1RM bench press, with an average increase from 214 lb to 221 lb. However, no acute effect on power was observed when jump squats at 30% of squat 1RM were performed after repetitions using different percentages of 1RM during the concentric and eccentric phases (Moore et al. 2007).
- Variable Findings: Studies have reported mixed results. For instance, some research suggests that accentuated eccentric DCER training can enhance maximal concentric power (Ojastro and Hakkinen 2009). In contrast, another study showed no significant difference in maximal concentric strength but a significant increase in concentric power (Moore et al. 2007).
- Comparative Strength Gains: Over seven consecutive training days, accentuated eccentric training was found to increase strength to a greater extent than normal DCER training (Hortobagyi et al. 2001). However, normal training, consisting of sets of 10 to 12 repetitions at approximately 60% of 1RM, resulted in similar gains in concentric 3RM and isokinetic (90 degrees per second) concentric strength. In contrast, accentuated eccentric training led to significantly greater gains in eccentric 3RM, isokinetic (90 degrees per second) eccentric, and isometric strength. These changes were attributed to neural adaptations over this short training period.
- Isokinetic Training: In a study comparing accentuated eccentric isokinetic training with training involving both concentric and eccentric phases, both methods were found to yield similar gains in concentric-only isokinetic (30 degrees per second) strength. This suggests that accentuated eccentric training can be as effective as traditional training with both concentric and eccentric phases (Godard et al. 1998).
- Training for Seniors: Research has shown that seniors can safely perform DCER eccentric accentuated training with various machine exercises (Nichols, Hitzelberger et al. 1995). In this study, the eccentric portion of repetitions had a higher percentage of 1RM compared to the concentric phase. While there was little advantage in strength gains after 12 weeks of training, the accentuated eccentric system was deemed safe for seniors.
- Mixed Results in Untrained Individuals: Studies involving untrained individuals have produced varied findings. In one study (Brandenburg and Docherty 2002), both traditional and accentuated eccentric training led to similar gains in 1RM strength for elbow flexors but accentuated eccentric training resulted in greater 1RM gains for elbow extensors. In another study (Yarrow et al. 2008), untrained men showed similar strength gains in the bench press and squat with either traditional or accentuated eccentric training, along with similar hormonal responses.
In conclusion, accentuated eccentric training has shown both potential and variability in its impact on strength gains. While it can result in acute increases in strength, especially when incorporated immediately before maximal efforts, its long-term effectiveness compared to traditional training methods remains a subject of ongoing research. The outcome may be influenced by factors like training experience, muscle group targeted, and the specific training protocol used.
Strength Gains and Accentuated Eccentric Training: A Holistic View
The previous discussion underscores several important findings regarding accentuated eccentric training and its impact on strength gains. To summarize:
- Effect of Resistance Levels: When less-than-normal 1RM is used in accentuated eccentric training, it does not appear to provide an advantage in strength gains over traditional training.
- Greater-Than-Normal 1RM: In contrast, when greater-than-normal 1RM is used during the eccentric repetition phase of accentuated eccentric training, it can lead to greater 1RM gains, especially in specific muscle groups such as elbow extensors. This suggests that the effectiveness of accentuated eccentric training may depend on the magnitude of resistance used during the eccentric phase.
- Supporting Evidence: This observation finds support in research conducted by Schroeder, Hawkins, and Jaque (2004) over a 16-week period, involving young women training with heavy negative-only (125% of 1RM for three sets of six repetitions) and light negative-only (75% of 1RM for three sets of 10 repetitions) training. Both groups experienced significant improvements in 1RM for all six exercises (20-40%). The heavy negative-only training group exhibited greater percentage gains in five of the six exercises, with the chest press showing significantly greater gains in 1RM (65% vs. 40%). This implies that heavy negative-only training can provide an advantage in maximal strength gains. Additionally, both groups saw significant increases in lean mass as measured by dual-energy X-ray absorptiometry, with the heavy negative-only training group experiencing a significantly greater gain.
- Eccentric-Only Training: Eccentric-only training has been shown to result in strength gains, and in some instances, these gains may surpass those of normal training. However, the majority of evidence suggests that there are typically no significant differences between eccentric-only and traditional training.
- Accentuated Eccentric Training: Studies have demonstrated that accentuated eccentric training, particularly among trained or moderately trained individuals, can lead to significant strength gains. This is especially notable when strength is measured in an eccentric manner. Moreover, accentuated eccentric training may have a potential advantage over normal resistance training, but the use of greater-than-normal 1RM in the eccentric phase of repetitions is a crucial factor.
- Muscle Group Variability: It’s important to note that not all muscle groups respond equally to accentuated eccentric DCER training, and the effectiveness of this training method can vary between muscle groups.
Optimal Eccentric Training: Unveiling the Factors
Achieving optimal results in eccentric training involves several factors, including resistance levels, exercise selection, and the number of repetitions. Here’s a comprehensive look at these elements:
Resistance Levels in Eccentric Training: Studies have reported increases in strength following eccentric-only DCER training using various resistance levels. Some of the reported resistance levels include:
- 120-180% of maximal isometric strength (Colduck and Abernathy 1997)
- 80% of the eccentric 1RM (Housh et al. 1998)
- 75% of concentric 1RM (Schroeder, Hawkins, and Jaque 2004)
- 100% of normal 1RM (Martin, Martin, and Morlon 1995)
- 120% of normal 1RM (Johnson et al. 1976)
- 125% of concentric 1RM (Schroeder, Hawkins, and Jaque 2004)
- 100% of 10RM (Smith and Rutherford 1995)
- 80% of 5RM (Reeves et al. 2009)
- 85-90% of 4- to 8RM (Vikne et al. 2006)
Additionally, maximal isokinetic eccentric-only muscle actions have also shown increases in strength (Hawkins et al. 1999; Hortobagyi et al. 1996; Seger, Arvidsson, and Thorstensson 1998). Furthermore, accentuated eccentric DCER training using 40 to 50% more resistance than in the concentric phase of repetitions (Hortobagyi et al. 2001) and 75% of 1RM in concentric and 110 to 120% of 1RM in eccentric repetition phases (Brandenburg and Docherty 2002), as well as accentuated eccentric isokinetic training using 40% more resistance than in the concentric phase of repetitions (Godard et al. 1998), have shown significant strength increases.
However, what constitutes the optimal eccentric resistance for training remains an open question. While Jones (1973) suggested that the optimal resistance is one that the trainee can lower slowly and stop at will, Johnson and colleagues (1976) claimed that a resistance of 120% of the DCER 1RM is optimal. But, studies have shown significant strength increases using resistance both greater than and less than 120% of the DCER 1RM. For example, eccentric strength, depending on the velocity of movement, can be greater than or at least equal to maximal isometric strength and reach up to 180% of maximal isometric strength (Colduck and Abernathy 1997).
This indicates that while resistance levels are important, the concept of an optimal eccentric resistance remains to be precisely defined. There might be a practical upper limit to the resistance that can be effectively used in eccentric training.
Number of Repetitions in Eccentric Training: Another practical question surrounding eccentric training is the number of repetitions needed to be performed in a heavy eccentric or accentuated eccentric manner to yield the desired results. One study conducted on highly trained competitive Olympic weightlifters suggests that as few as 25% of the total number of DCER repetitions need to be performed in an accentuated eccentric manner to achieve greater strength gains than normal DCER training (Häkkinen and Komi 1981). However, it’s crucial to note that these findings are most applicable to highly trained resistance athletes.
In conclusion, optimal eccentric training involves carefully considering resistance levels, exercise selection, and the number of repetitions. While specific optimal resistance levels are yet to be fully elucidated, it’s clear that resistance exceeding the standard 1RM can be beneficial. Furthermore, understanding the training requirements for different populations, from highly trained athletes to individuals new to resistance training, is essential for optimizing eccentric training outcomes.
Motor Performance and Body Composition Changes in Eccentric Training
Eccentric training and accentuated eccentric training have the potential to influence motor performance and body composition. Here’s a comprehensive exploration of the effects and outcomes associated with these training methods:
Motor Performance:
- Strength Gains: Eccentric training, including accentuated eccentric training, has shown to increase isometric, concentric, and eccentric strength. These strength improvements can potentially enhance motor performance abilities.
- Vertical Jump: The effects of eccentric training on vertical jump ability are mixed. Some studies have reported an increase in vertical jump ability, while others found no significant changes. This discrepancy suggests that the impact of eccentric training on vertical jump performance may vary depending on factors like training protocols and the characteristics of the individuals being studied.
- Tennis Serve Velocity: The influence of eccentric training on tennis serve velocity remains uncertain. Some studies have reported no significant change in tennis serve velocity resulting from isokinetic eccentric training of the shoulder and arm musculature, while others have shown a significant increase. However, in the latter case, the increase was not significantly different from that achieved through isokinetic-concentric training. This implies that the specific impact of eccentric training on tennis serve velocity is still a subject of ongoing investigation.
- Power Enhancement: Acutely, accentuated eccentric training has demonstrated the potential to increase power in the concentric phase of exercises such as the bench press. This suggests that accentuated eccentric training can positively affect motor performance, particularly in tasks involving rapid power generation. However, the overall impact of eccentric training on motor performance remains an area of active research.
Body Composition Changes:
- Muscle Protein Synthesis: Eccentric-only and concentric-only muscle actions have both been shown to increase muscle protein synthesis and muscle protein breakdown, leading to a net increase in muscle protein synthesis. These changes have been observed in untrained subjects, with no significant differences based on the type of muscle action.
- Fat-Free Mass and Muscle Hypertrophy: Eccentric training has been associated with increases in limb circumference, muscle cross-sectional area, and muscle thickness. These changes indicate muscle hypertrophy and are typically associated with an increase in fat-free mass. However, these increases in size are not consistently different from those achieved through concentric or coupled concentric-eccentric training. The response to eccentric training may vary depending on factors such as the specific training protocols used in the studies.
- Muscle Fiber Cross-Sectional Area: Eccentric-only training has been shown to increase the cross-sectional area of both type I and type II muscle fibers. In contrast, concentric-only training has not consistently resulted in significant changes in these measures. The specific responses of muscle fibers may vary depending on the type and velocity of eccentric training.
Postexercise Soreness in Eccentric Training
Eccentric training, particularly with greater-than-1RM concentric strength or maximal eccentric actions, is associated with a unique phenomenon known as postexercise soreness or delayed-onset muscle soreness (DOMS). This condition can result in greater soreness compared to isometric, concentric, or concentric-only isokinetic training. Here is a comprehensive discussion of the development, characteristics, and potential adaptations related to postexercise soreness in eccentric training:
Development and Characteristics of DOMS:
- Greater Soreness: Eccentric training, especially when using resistance greater than the concentric 1RM, tends to produce more significant postexercise soreness. The soreness is more intense than that experienced after isometric, concentric, or concentric-only isokinetic training.
- Onset and Duration: DOMS typically begins around eight hours after an eccentric exercise session, peaks at two to three days post-exercise, and can persist for 8 to 10 days. The onset of soreness can vary among individuals, but it follows a consistent pattern of peak intensity and gradual reduction.
- Strength Reduction: Strength levels may decrease for up to 10 days following an eccentric exercise bout. This strength reduction corresponds with the period of maximal soreness.
- Protective Effect: Surprisingly, one bout of eccentric exercise can provide protection against excessive soreness caused by subsequent eccentric exercise sessions for an extended period, which may last up to seven weeks for untrained or novice weight training individuals. This protective effect can also occur with low-volume and low-intensity eccentric training.
- Velocity-Dependent Soreness: Performance of eccentric training at one velocity may decrease muscle soreness caused by an exercise bout at another eccentric velocity. This indicates that the specific velocity of eccentric training may influence the subsequent soreness.
- Threshold Resistance: There is some indication that eccentric actions must be performed with a resistance greater than the concentric 1RM to induce significant soreness. However, research has shown that maximal eccentric actions result in greater muscle damage than eccentric actions performed with 50% of maximal isometric force.
- Factors Involved: Various factors contribute to DOMS, including edema, inflammation, sarcomere length alterations, impaired muscle glycogen resynthesis, muscle spasm, and enzymes leaking out of muscle fibers due to membrane damage. The complex interplay of these factors results in muscle soreness and strength loss following eccentric exercise.
Adaptations and Mitigation of Soreness:
- Protection from Soreness: Repeated bouts of eccentric exercise may lead to adaptations that protect against excessive muscle soreness in subsequent exercise sessions. These adaptations may involve increased activation of type I muscle fibers and the addition of sarcomeres in series to avoid damage to the muscle fibers.
- Adaptations for Protection: The exact mechanisms responsible for adaptations that reduce soreness remain unclear. However, there is evidence to suggest that these adaptations exist and are triggered by repeated eccentric exercise, contributing to the reduction of soreness in successive bouts.
Comparison of Different Resistance Training Types
Comparing various types of resistance training is a challenging task, as multiple factors come into play when determining the most effective training for specific physiological adaptations. Several difficulties arise in conducting such comparisons, including the issue of specificity of training, the challenges in equating total training volume and work, the duration of training sessions, as well as the differences in subject training status and the duration of the studies. Here’s a comprehensive discussion of these difficulties and considerations:
Specificity of Training and Strength Gains:
- The principle of specificity plays a crucial role in resistance training. When training and testing are conducted using the same type of resistance equipment, substantial increases in strength are typically observed.
- In contrast, if training and testing involve different types of equipment, the increase in strength is often significantly less or even non-existent.
- Ideally, strength should be tested using various types of muscular actions to evaluate training specificity and its potential carryover to other types of muscular actions.
Challenges in Equating Training Variables:
- Comparing different resistance training types is further complicated by discrepancies in equating variables like total training volume (sets and repetitions), total work (repetitions × resistance × vertical distance the weight is moved), and the duration of training sessions.
- These differences make it challenging to compare training types fairly and conclusively demonstrate one type’s superiority over another.
Study Design Difficulties:
- Several study design difficulties hinder the generalization of results to various populations. These difficulties include the training status of subjects and the fact that some studies only focus on training one muscle group.
- Applying results from training one muscle group or exercise to another can be problematic because not all muscle groups respond with the same magnitude or timeline of adaptations.
- Most comparisons involve novice subjects with relatively short training durations (typically 10-20 weeks), making generalization to highly trained individuals and long-term training (spanning years) challenging.
Illustrative Study:
- An example is provided to illustrate these difficulties. In a particular study, subjects underwent eight weeks of training, using both isometric and dynamic constant external resistance (DCER) regimes. Results showed varying outcomes based on the muscle groups tested and the type of training.
- Isometric training led to a 0% increase in isometric elbow flexion force but a 35% increase in isometric elbow extension force.
- DCER training resulted in a 9% increase in isometric elbow flexion force and a 16% increase in isometric elbow extension force.
- Thus, the effectiveness of isometric and DCER training appeared to vary depending on the muscle group and type of DCER regime employed.
Testing Specificity:
- Testing specificity must be taken into account when comparing two types of resistance training within the same general category, such as DCER. The specific muscle groups targeted and the nature of the training regimes can affect the results.
Efficacy of Training Programs:
- The most critical factor when comparing training types is the efficacy of the training programs in bringing about physiological adaptations. The optimal program design can significantly influence results, and if programs are not optimal, conclusions drawn from studies should be viewed with caution.
In conclusion, comparing different types of resistance training poses challenges due to the specificity of training, disparities in training variables, study design difficulties, and varying efficacy of training programs. Although certain conclusions can be drawn from these comparisons, it’s essential to approach the results with caution and recognize that further research is needed to provide more comprehensive insights into the effectiveness of various training modes.
Comparison of Various Resistance Training Types
In the realm of resistance training, there are different approaches and methods, each with its unique characteristics and effects on strength and motor performance. This section delves into the comparisons between various resistance training types and their impact on strength gains, isometric training, DCER training, variable resistance training, and concentric isokinetic resistance training.
Isometric vs. Dynamic Constant External Resistance (DCER) Training:
- The effectiveness of isometric training versus DCER training often follows a pattern of test specificity. When isometric testing methods are employed, isometric training tends to show superiority, and when DCER testing (1RM) is used, DCER training emerges as the more effective approach.
- It’s essential to note that some studies have demonstrated that DCER training can result in greater increases in isometric force compared to isometric training.
- The effectiveness of isokinetic testing for strength gains is inconclusive, with results varying depending on testing velocities.
- A review of the literature suggests that well-designed DCER programs are generally more effective than standard isometric programs for increasing strength. However, both training modes can lead to muscle hypertrophy, and there’s no clear favoring of one over the other for this parameter.
Isometric vs. Variable Resistance Training:
- There is a lack of direct studies comparing isometric training with variable resistance training. However, based on available knowledge, hypotheses can be made.
- Motor performance improvements are a key aspect of consideration. Isometric training at one joint angle does not consistently enhance dynamic motor performance, while variable resistance training has shown the potential for such improvements. Therefore, variable resistance training may be superior to isometric training when an increase in motor performance is desired.
Isometric vs. Concentric Isokinetic Resistance Training:
- Comparisons of isometric and concentric isokinetic training primarily exhibit a pattern of test specificity, with outcomes varying depending on the type of testing employed.
- Direct comparisons have mainly used relatively slow-velocity isokinetic training (up to 30 degrees per second).
- The effectiveness of isometric training compared to isokinetic training depends on the muscle group tested and the specific conditions. For some muscle groups, isometric training results in superior isometric force improvements, while for others, isokinetic training leads to better results.
- Similar to other comparisons, test specificity plays a role in the outcomes.
- Isokinetic training has demonstrated its ability to improve motor performance, while isometric training at one joint angle does not consistently show the same effect.
- Both isometric and isokinetic training can lead to significant muscle hypertrophy, with no clear superiority of one mode over the other.
Isometric vs. Eccentric Resistance Training: A Comparative Analysis
This section provides a comparative analysis between isometric training and eccentric training, particularly focusing on free weights or conventional resistance training machines. The aim is to understand the differences and similarities in terms of strength gains, isometric strength, and motor performance improvement.
Isometric vs. Eccentric Training for Strength Gains:
- When assessed using isometric measurements, the studies reviewed indicate that there are no significant differences in strength gains between isometric and eccentric training.
- For example, one study compared training the elbow flexors and knee extensors exclusively with either isometric or eccentric training. Both training methods involved ten maximal five-second actions per day. The results showed that isometric training led to improvements in isometric strength, with 13.8% increase for males in elbow flexion and 10% in knee extension. In contrast, eccentric training resulted in 8.5% improvement for males in elbow flexion and 14.6% in knee extension. For females, similar trends were observed with no substantial difference between isometric and eccentric training.
- Another study that involved training subjects’ knee extensors three times per week for six weeks also concluded that isometric and eccentric training improved isometric knee extension force with comparable gains.
Isometric vs. Eccentric Training for Motor Performance:
- The reviews suggest that isometric training at one joint angle does not typically lead to increased motor performance.
- In contrast, the impact of eccentric training on motor performance is less clear. Some studies have reported motor performance increases following eccentric training, while others have shown no significant change.
Overall Assessment:
- In summary, based on the studies available, isometric training and eccentric training with free weights or conventional resistance machines appear to have similar effects on isometric strength gains when measured isometrically.
- When it comes to motor performance improvement, the superiority of one training type over the other remains unclear, with mixed results reported.
- It’s important to note that the choice between isometric and eccentric training may depend on specific goals, preferences, and the particular muscle groups being targeted.
This comparative analysis highlights the value of considering individual objectives and requirements when selecting between isometric and eccentric resistance training.
Dynamic Constant External Resistance vs. Variable Resistance Training: A Comparative Analysis
This section presents a comprehensive comparative analysis between Dynamic Constant External Resistance (DCER) training and Variable Resistance training, with a focus on strength gains, exercise-specificity, and body composition changes. The aim is to understand the differences and similarities between these two training methods.
Strength Gains – Variable Results:
- Comparisons of strength gains as a result of DCER and variable resistance training have yielded mixed results.
- In one study after 20 weeks of training, variable resistance training demonstrated a clear superiority over DCER training in terms of 1RM free weight bench press ability, with gains of 29.5% compared to 14% in the DCER group.
- Another bench press comparison indicated a specificity of training. Both training types showed significantly greater increases in 1RM over the other when tested on the type of equipment on which training was performed.
- In contrast, leg press strength exhibited test specificity for the training methods. After 10 weeks of training, a variable resistance group improved significantly when tested with variable resistance equipment, but only minimally when tested with DCER methods. The DCER group showed the opposite pattern of results.
- Another study showed that after 12 weeks, both DCER and variable resistance training improved strength significantly when tested on the equipment they trained on, with no clear superiority of one over the other.
Isometric Strength – Comparable Gains:
- Studies have shown that DCER and variable resistance training result in similar gains in isometric strength. For instance, one study found no significant difference in isometric knee extension strength gains after 10 weeks of training using both methods.
- These results indicate that both DCER and variable resistance training yield comparable improvements in isometric strength, regardless of the specific knee angle tested.
Effect on Vertical Jump Ability – Equipment-Dependent:
- The type of variable resistance equipment or training program used can impact the effectiveness of both DCER and variable resistance training. For example, a study demonstrated that both DCER (free weight) and increasing lever-arm variable resistance training led to significantly greater increases in vertical jump ability than cam-type variable resistance training.
- This suggests that the superiority of one training type over the other might be influenced by the choice of variable resistance equipment or the specific training program employed.
Body Composition Changes – Similar Outcomes:
- The available evidence indicates that body composition changes resulting from DCER and variable resistance training are of similar magnitude.
- Comparative studies lasting 10 weeks and 12 weeks have shown no significant difference between the two training methods in changes related to percent fat, fat-free mass, total body weight, and limb circumferences.
- These findings suggest that DCER and variable resistance training have similar effects on body composition changes.
In conclusion, the comparison of DCER and variable resistance training reveals mixed results in terms of strength gains, with factors such as equipment choice and exercise specificity influencing outcomes. However, both training methods appear to result in comparable improvements in isometric strength and similar body composition changes. The choice between these two methods may depend on specific training goals, preferences, and the equipment available.
Concentric vs. Eccentric Resistance Training: A Comprehensive Comparison
This section presents a thorough comparative analysis between concentric and eccentric resistance training. It explores different aspects, including strength gains, muscle-specific actions (such as DCER and isokinetic training), contraction mode specificity, and their impact on motor performance and body composition.
DCER Training: No Significant Difference in Strength Gains:
- A review of comparative studies indicates that there is no significant difference in strength gains between concentric and eccentric training when using Dynamic Constant External Resistance (DCER) equipment.
- For instance, strength gains tested with DCER exercises like elbow curls, arm presses, knee flexions, and knee extensions after six weeks of training are not significantly different between these two training types.
- After 20 weeks of training, both concentric and eccentric DCER training showed little advantage in isometric or isokinetic strength gains, indicating comparable outcomes.
Eccentric-Only DCER Training vs. Concentric-Only DCER Training: Different Results:
- However, studies have shown that eccentric-only DCER training can result in significantly greater eccentric 1RM strength gains compared to concentric-only DCER training.
- A comparison of various squat training methods revealed that training with both eccentric and concentric actions led to significantly greater gains in 1RM squat ability, suggesting that both types of muscle actions may be necessary for maximal strength gains.
Isokinetic Training – Variable Results:
- When comparing concentric-only and eccentric-only isokinetic training, short-term training periods have shown no significant difference in maximal concentric, eccentric, or isometric strength increases.
- However, contraction mode specificity has been observed in isokinetic training, where training in concentric-only and eccentric-only muscle actions at different speeds and resistances has demonstrated varying results. This specificity is not always consistent.
- In some cases, fast eccentric-only training has shown greater strength gains than slow eccentric-only or concentric-only isokinetic training.
Motor Performance Impact – Inconclusive:
- The effect of isokinetic concentric-only and eccentric-only training on motor performance, such as tennis serve velocity, is inconclusive. Different studies have reported mixed results, with some showing that both eccentric and concentric training can improve serve velocity, while others indicate eccentric training as more beneficial.
- Overall, the influence of these training methods on motor performance appears to vary, and no clear superiority has been established.
Impact on Body Composition:
- Eccentric training does bring about increases in motor performance and changes in body composition, such as increased fat-free mass. However, the changes appear not to be significantly different from those resulting from other types of muscle actions or training types.
- Both concentric-only and eccentric-only isokinetic training have shown an ability to increase muscle and muscle fiber cross-sectional area, indicating that both can affect body composition by increasing fat-free mass.
Considerations for Eccentric-Only Training:
- Eccentric-only training may lead to post-exercise soreness, especially during the initial weeks of training. Thus, it’s recommended to incorporate eccentric-only training gradually into a workout program to minimize muscle soreness.
Dynamic Constant External Resistance vs. Isokinetic Resistance Training: A Comprehensive Comparison
This section provides a comprehensive comparison between Dynamic Constant External Resistance (DCER) and isokinetic resistance training, covering various aspects such as strength gains, muscle-specific actions, contraction mode specificity, biomechanical comparisons, and their impact on motor performance and body composition.
Strength Gains – No Clear Superiority:
- Studies comparing DCER and concentric-only isokinetic resistance training indicate no clear superiority of either type over the other.
- Various studies have reported different results in terms of strength gains. For instance, after eight weeks of training, isokinetic training showed better results in some cases, while DCER training yielded superior outcomes in others.
- Factors such as training protocols, resistance levels, and testing methods can influence the results, making it challenging to determine a clear winner in terms of strength gains.
Testing Specificity:
- Both DCER and isokinetic training have shown testing specificity in certain studies. This means that the training method that matches the testing conditions often results in more significant gains.
- For example, DCER and isokinetic training have demonstrated significant increases when tested in a manner consistent with their respective training types.
Biomechanical Comparison:
- A biomechanical comparison of free weight and isokinetic bench pressing indicated some similarity. Subjects performed both free weight and isokinetic bench presses at different percentages of their 1RM, and no significant difference in maximal force was found between the two.
- This suggests that, at least in the context of force production during a major portion of an exercise movement, free weights can affect muscles in a manner similar to isokinetic devices.
Impact on Motor Performance:
- Both DCER and isokinetic training can increase motor performance ability similarly, as demonstrated in a comparison of two-legged leg press training. This study showed no significant difference in one-legged jumping ability between the two training methods.
Changes in Body Composition:
- Both DCER and isokinetic training have shown similar changes in body composition. The magnitude of changes in percent fat, fat-free mass, and total body weight did not significantly differ between the two methods.
Isokinetic vs. Variable Resistance Training: A Comprehensive Comparison
This section provides a comprehensive comparison between Isokinetic and Variable Resistance Training, focusing on factors such as testing specificity, strength gains, motor performance benefits, and body composition changes.
Testing Specificity:
- Comparisons of Isokinetic and Variable Resistance Training demonstrate testing specificity. This means that the results often favor the training method that matches the testing conditions.
- A study compared slow- and fast-speed concentric-only isokinetic training with variable resistance training for the knee extensors and flexors. Slow-speed isokinetic training was performed at velocities of 30, 60, and 90 degrees per second, while fast-speed isokinetic training had velocities of 180, 240, and 300 degrees per second.
- Variable resistance training initially consisted of three sets of 10 repetitions at 80% of 10RM with resistance increased as strength improved.
- In measures of strength, isokinetic training showed a consistent pattern of test speed specificity, while variable resistance training displayed consistent increases in knee flexion regardless of the test criterion. However, knee extension showed large increases in isometric force only.
- Leg press results also illustrated testing specificity, with variable resistance and slow-speed isokinetic training showing similar and larger gains compared to fast-speed isokinetic training.
Motor Performance Benefits:
- The study compared the motor performance benefits of isokinetic and variable resistance training.
- Fast-speed isokinetic training demonstrated greater increases in all three motor performance tests compared to the other two training types.
- Variable resistance and slow-speed isokinetic training groups showed similar changes in the motor performance tests.
- These results indicate that fast-speed isokinetic training may be superior to slow-speed isokinetic and variable resistance training in the context of motor performance improvement.
Body Composition Changes:
- Body composition changes due to isokinetic and variable resistance training appear to be similar, although minimal data are available to draw comprehensive conclusions.
- The available data suggest that both training types bring about similar changes in body composition.